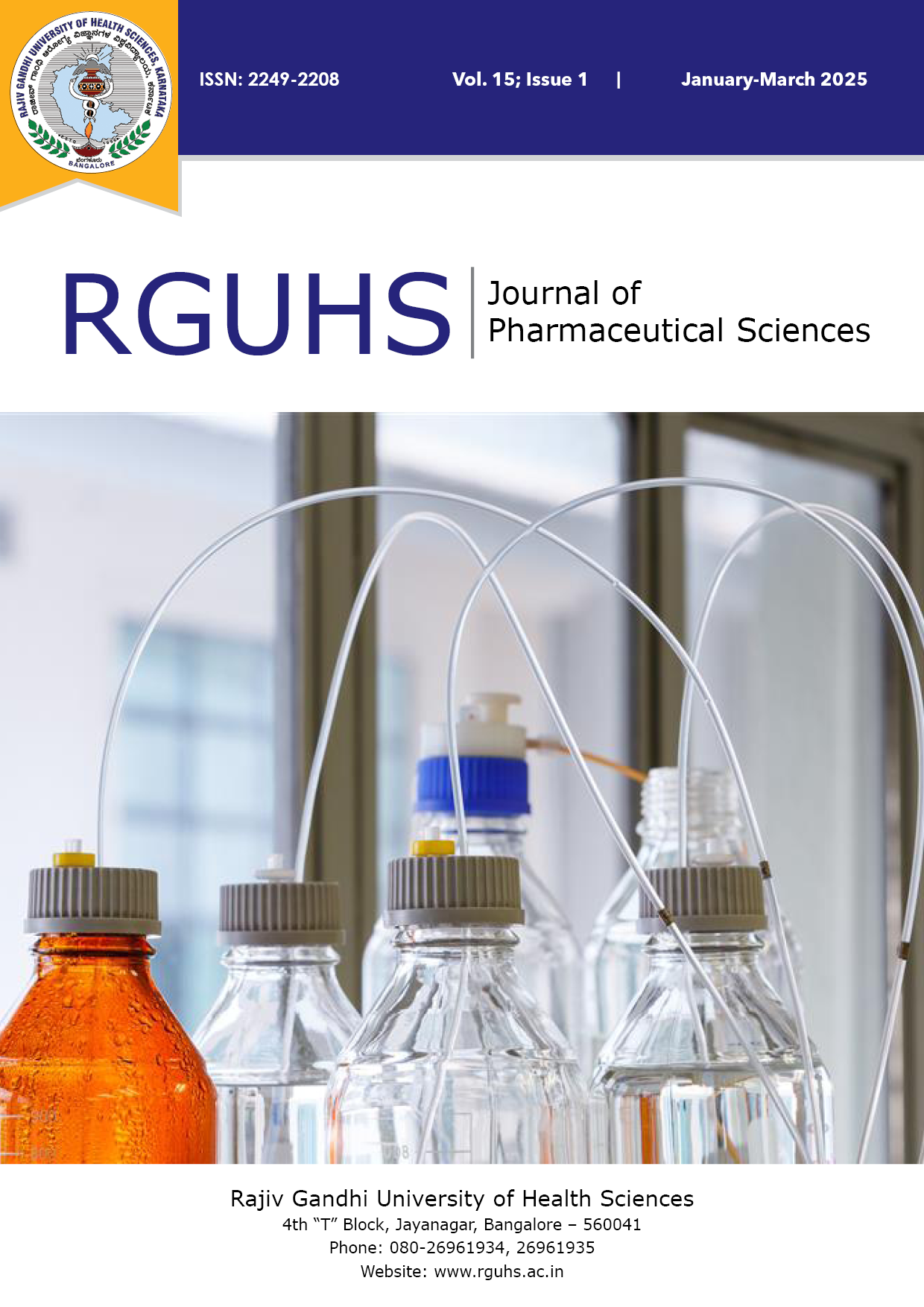
RJPS Vol No: 15 Issue No: 1 eISSN: pISSN:2249-2208
Dear Authors,
We invite you to watch this comprehensive video guide on the process of submitting your article online. This video will provide you with step-by-step instructions to ensure a smooth and successful submission.
Thank you for your attention and cooperation.
1Department of Pharmaceutical Chemistry, Government College of Pharmacy, Bengaluru, Karnataka, India
2Dr. Chaluvaraju K C, Associate Professor, Department of Pharmaceutical Chemistry, Government College of Pharmacy, Bengaluru, Karnataka, India.
3Research and Development, Rajiv Gandhi University of Health Sciences, Karnataka, Bengaluru, India
*Corresponding Author:
Dr. Chaluvaraju K C, Associate Professor, Department of Pharmaceutical Chemistry, Government College of Pharmacy, Bengaluru, Karnataka, India., Email: chaluvarajukc@gmail.com
Abstract
Background: Syzygium aromaticum L. buds are the primary source of eugenol (2-methoxy-4-(prop-2-en-1-yl) phenol), a naturally occurring phenylpropanoid. It exhibits anticancer, antioxidant, antibacterial, antifungal, and anti-inflammatory properties. Its quantification is essential for quality control in pharmaceutical and industrial applications.
Objectives: This study aimed to develop and validate a rapid, efficient, and precise high-performance thin-layer chromatography (HPTLC) method for estimating eugenol isolated from S. aromaticum L. buds.
Methods: The structure of Eugenol was confirmed using thin-layer chromatography (TLC), Fourier-transform infrared spectroscopy (FTIR), proton nuclear magnetic resonance (1H NMR) and gas chromatography-mass spectrometry (GC-MS). HPTLC analysis employed a mobile phase comprising toluene, ethyl acetate, and glacial acetic acid in a 7.8:2.2:0.1 (v/v/v) ratio. Densitometric scanning was performed in the absorbance mode at 282 nm. The method was validated by International Conference on Harmonization (ICH) guidelines.
Results: The developed HPTLC method was validated for specificity, precision, linearity, accuracy, sensitivity, and robustness.
Conclusion: This validated HPTLC method provides a reliable and precise approach for routine quality control of eugenol in raw materials and formulations. It ensures product quality, safety, and consistency in pharmaceuticals, cosmetics, essential oils, and industrial applications. The method supports regulatory compliance and facilitates innovation in the therapeutic and commercial use of eugenol-based products.
Keywords
Downloads
-
1FullTextPDF
Article
Introduction
Eugenol is a phenylpropanoid found in plants such as clove buds (Syzygium aromaticum L.), black pepper (Piper nigrum), cinnamon bark and leaves (Cinnamo-mum spp.), ginger (Zingiber officinale), tulsi leaves (Ocimum sanctum), and thyme (T. vulgaris) (Figure 1).1,2 Clove oil is the primary source of eugenol. It contains 84-95% phenols, including eugenol, eugenol acetate, α and β caryophyllenes, and trace amounts of alcohols, ketones, and esters.3
Eugenol has numerous pharmacological properties, including anticancer activity, antioxidant, antibacterial, antifungal, and anti-inflammatory properties.4-15 Eugenol is a target molecule for structural modifications to produce pharmaceutically active substances due to its broad spectrum of biological activity.16,17
Traditional analytical techniques for volatile oil estimation include specific gravity, microscopic inspection, refractive index, optical rotation, microscopic inspection, fluorescence, and solubility. These non-specific methodologies provide minimal qualitative information. Using advanced chromatographic techni-ques to identify and quantify these active substances is highly beneficial for the quality control of medicines derived from medicinal plants and their essential oils.
The technique most frequently used to analyse volatile components is gas chromatography. The GC method is highly complex, time-consuming, and skill-intensive. For polar aromatic compounds, TLC and HPLC work better. Nonetheless, HPTLC offers several benefits due to its capacity to separate samples and simultaneously tolerate crude extracts. With its high sample throughput and in-situ spectrum recording abilities, it is also inexpensive and provides more straightforward and reliable component identification and quantification.18 Given the above facts and the paucity of literature on the quantification of eugenol by HPTLC, a sincere effort has been made to develop and validate the HPTLC method for estimation of eugenol isolated from S. aromaticum L. in the laboratories of the Government College of Pharmacy, Bengaluru.
Materials and Methods
Plant material and standard
Clove buds were purchased from M/S Amruth Kesari, Bengaluru, and authenticated by the Regional Ayurveda Research Institute for Metabolic Disorder, Bengaluru (Authentication/SMPU/CARI/BNG/2020-21/1827). Poornayu Research Labs, Bengaluru, provided a working standard of eugenol as a gift sample.
Reagents and solvents
Methanol HPLC grade, 0.45 μm nylon syringe filter from Merck. Dichloromethane, toluene, ethyl acetate, glacial acetic acid, and anhydrous sodium sulphate purchased from SD fine chemicals were used in the present study.
Instruments
Evaporation and concentration in vacuo were performed using a rotary evaporator (Bucchi). The FT-IR spectrum was measured on a Bruker (Alpha II ATR) instrument. 1HNMR spectra were recorded on a 400 MHz Avance Bruker instrument. GCMS was recorded on the Shimadzu-QP2020 NX single quadrupole system. CAMAG HPTLC System with WINCAT’s software (version V 1.4.4), CAMAG Linomat V autosampler, CAMAG TLC visualizer, CAMAG TLC densitometer III, and CAMAG 20 cm x 10 cm twin trough glass chamber. Aluminium silica gel 60F254 plates (20 × 20 cm), 0.25 mm thickness (MERCK) were used for the estimation of eugenol.
Extraction and Isolation of Eugenol from clove buds
Fifteen grams of freshly grounded clove buds were weighed precisely and placed in 500 mL of RBF. Clevenger's apparatus was used for hydrodistillation, and extraction was performed at temperatures ranging from 50 to 70°C for a set duration. The distillation process was stopped when the distillate became colorless. Dichloromethane (3x50 mL) was used to extract the collected emulsion. The aqueous layer was discarded, and the dichloromethane layer was dried and filtered over anhydrous sodium sulphate. A brown oil was formed when the solvent was removed using a rotary evaporator. The FT-IR analysis revealed a mixture of eugenol and carbonyl compounds. The crude oil was column chromatographed several times using the n-hexane: ethyl acetate (7:3 v/v) ratio as the mobile phase and silica gel mesh size of 230-400 as the stationary phase. The collected sample was characterized using TLC, FTIR, 1H NMR and GC-MS; the fraction was identified as eugenol.19,20
Method development
Preparation of standard solution
A standard solution was prepared by accurately weighing 80 mg of eugenol standard and transferring it to a clean, dry 100 mL volumetric flask. The solution was then dissolved and diluted with methanol to obtain a concentration of 800 μg/mL.
Preparation of sample solution
Accurately weighed 80 mg of the isolated eugenol sample and transferred to a clean, dry 100 mL volumetric flask. Dissolved and diluted with methanol to obtain a concentration of 800 μg/mL.
The 5 μL of standard and sample aliquots were spotted as bands (8 mm width) with a Camag Linomat V autosampler onto an aluminium precoated silica gel 60F254 plate (20 x 10 cm). The application rate was constant (0.1 μL/s), and the distance between the two bands was 6 mm.
The mobile phase for analysis is chosen toluene:ethyl acetate:glacial acetic acid (7.8:2.2:0.1 v/v/v), and no immiscibility issues were observed. Linear ascending development was carried out in a twin-trough glass chamber saturated with the mobile phase. The mobile phase optimum chamber saturation time was 30 minutes. The plates were developed to a height of 7 cm under chamber saturation conditions. TLC plates were dried in an air current using an air dryer after development and scanned in absorbance mode at 282 nm using a Camag TLC scanner and Wincat software with a silt dimension of 6 x 0.45 mm and a scanning speed of 20 mm/s. A deuterium lamp was used as a radiation source.21,22
Method validation
The method was validated in accordance with ICH Q2 (R2) guidelines. The method specificity was determined; there was no interference from the blank or other matrix of the sample, and well-defined peaks obtained indicated the method specificity. Linearity was investigated in triplicate by applying standard solution concentrations ranging from 120 to 320 μg/mL to the TLC plate in the 0.8-6.4 μg/band range. The regression square equation was generated by plotting peak area versus concentration. The LOD and LOQ were found to be 0.3 μg/band and 1.0 μg/band, respectively, based on the standard calibration curve.
Determined method precision by spotting four μg/band of the standard and sample solution intraday, and the studies were also repeated inter-day using freshly prepared standards and samples, and the peak area and RSD values were calculated. The developed method accuracy was determined by spotting samples at three levels in triplicate (80, 100, and 120%) at concentrations of 3.2, 4.0, and 4.8 μg/bands, respectively, and calculating percentage recoveries. The method robustness was validated by small, deliberate changes in mobile phase composition (7.6:2.4:0.1% v/v/v to 7.8:2.0:0.1% v/v/v) and detection wavelength changes of ±2 nm by spotting four μg/band of the standard and sample solutions, and the % RSD was calculated.23
Literature comparison
The performance of the sustainable HPTLC method for estimating eugenol was compared to various analytical techniques reported in the literature. Table 1 summarizes the validation parameters of the present HPTLC technique versus those of other analytical methods for eugenol estimation.
The linearity range, accuracy, and precision of the sustainable HPTLC method were compared to the reported techniques. The GC-FID method for eugenol quantification had a linearity range of 10.0-220.0 μg/mL, accuracy of 100.11-101.49%, and precision of 0.97%, less favourable than the HPTLC method.24 Similarly, an HPLC method with a linearity range of 6.0--125.0 μg/mL exhibited accuracy values of 99.15-101.48% and precision of 0.56%, which were still inferior to the sustainable HPTLC method. 24 Another HPLC method reported a linearity range of 6.25-100 μg/mL with accuracy ranging from 96.80-99.56%, but its precision was slightly lower at 1.81%.25
The UPLC technique showed superior linearity with a range of 10-100 ng/mL, but its accuracy and precision were comparable to those of the HPTLC method.26 Based on these findings, the present sustainable HPTLC technique demonstrates high sensitivity and suitability for eugenol estimation compared to other reported methods.
While advanced methods like UPLC, GC-MS, and LC-MS offer high sensitivity and accuracy, they require complex instrumentation and skilled operators, which makes them less accessible for routine analysis, especially in resource-limited settings. Additionally, these methods often involve expensive reagents, solvents, and equipment, which can be cost-prohibitive for large-scale or routine quality control applications. In contrast, the validated HPTLC method presented in this study is simple, cost-effective, and robust, making it an ideal alternative for routine quantification and quality control of eugenol in both research and commercial applications.
Results
Eugenol was isolated from S. aromaticum L. and characterized by FTIR, 1H NMR, and GCMS, as depicted in Figure 2.
The validated method demonstrated high specificity, with no interference from the blank or sample matrix at the retention time of eugenol (Figure 3A). The precision of the HPTLC method was determined by spotting four μg/band of the standard and sample solutions (Figure 3B, 3C, and 3D). The established concentration range observed was 120 to 320 μg/mL on the TLC plate in the range 0.8-6.4 μg/band, and data from the regression line (Y=1637.7x 1379.4). With an R2 value of 0.9970, ensured significant linearity between the response and analyte concentration (Figure 3E).
The precision measurements were given as a percentage of RSD. The calculated RSD of repeatability, intermediate precision, and method precision was determined to be < 2%, indicating that the procedure is precise. The accuracy of this approach can be seen in the recovery percentage, which ranged from 99.82 to 98.20% for 90--110%. Standard deviation, RSD and Rf values did not significantly alter despite deliberate changes to the method parameters, highlighting the method's robustness (Table 2).
Discussion
Clove oil is extracted from the clove buds of S. aromaticum L. using the hydrodistillation method by Clevenger apparatus. The distillate was extracted with dichloromethane, and the aqueous layer was discarded. The organic layer dried and was filtered over anhydrous sodium sulphate. The removal of the solvent in a rotary evaporator produced a brown-coloured oil. The FT-IR analysis revealed a mixture of eugenol and carbonyl compounds. Therefore, the crude oil was column chromatographed on silica gel several times, and the main fraction was collected and analyzed. TLC was carried out using an n-hexane: ethyl acetate (7:3% v/v). The Rf value was found to be 0.80. Its FT-IR spectrum was identical to that of pure eugenol, revealing (cm-1): 3511 (-OH str.), 3076 and 3004 (=CH aromatic), 2975, 2938, 2842 (-CH str., aliphatic), 1638 (C=C olefinic), 1607, 1510, 1463 (C=C aromatic), 1431 (CH2, aliphatic), 1366 (-CH3), 1265, 1231 (C-O str.), its 1H NMR (CDCl3, 400 MHz) showed (δ): 3.309-3.325 (d, CH2, benzylic), 3.870 (s, CH3O), 5.036- 5.094 (m,=CH2, allylic group), 5.488 (s, OH phenolic), 5.899- 6.000 (m, -CH=, olefinic), 6.674- 6.690 (m, H-3 + H-5), 6.832- 6.853 (d, H-6, aromatic ring) and GC-MS (E.I.) showed m/z: 164 and retention time at 20.283 min. Based on these findings, this fraction was identified as eugenol. The isolated eugenol oil is clear to pale yellow in colour and has a 78% yield.
The initial HPTLC study was performed by spotting eugenol standard solution on the plates with 2 μL, 5 μL and 6 mm width band. The mobile phase was tried with various solvents such as toluene, methanol, benzene, ethyl acetate, glacial acetic acid, acetonitrile, acetone, and others to develop the plates. Based on this observation, different toluene: ethyl acetate: glacial acetic acid ratios were tested. The toluene: ethyl acetate: glacial acetic acid (7.8:2.2:0.1% v/v/v) mixture was chosen as the mobile phase for analysis after several trials, and no immiscibility issues were observed.
Comparing the real-time spectra of the standard eugenol with test samples that were separated under the same conditions allowed for the confirmation of the method specificity. A strong correlation between the sample and standard was evident in the overlay spectra at the spot’s peak start, apex, and end, demonstrating the method specificity. Over a wide concentration range, the calibration curve for analyte quantification was investigated. Peak area vs. concentration plots were linear in the 0.8-6.4 μg/band range, with a correlation coefficient (R2) of 0.9970. The typical regression equation was Y = 1637.7 x 1379.4. LOD and LOQ were determined using the calibration curve to be 0.3 and 1.0 μg/band, respectively. The intra-day and inter-day precision of 4.0 μg/band were investigated using tests performed on the same and different days. The method was precise, as evidenced by the low RSD values.
Recovery studies were conducted at three levels (80, 100, and 120%), with average recoveries of 99.82, 99.37, and 98.20%. The average percentage recoveries and low % RSD values demonstrate the method's reliability and reproducibility. Slight adjustments to the mobile phase composition and variations in wavelength had no impact on the chromatography.
The method's robustness was demonstrated by its ability to tolerate minor changes in the mobile phase composition and wavelength without affecting the results (Table 2), highlighting its suitability for high-throughput commercial applications.
The method’s specificity is advantageous for distinguishing eugenol from other compounds in clove oil, ensuring reliable quality control testing. Its scalability, allowing for the simultaneous analysis of multiple samples on a single HPTLC plate, enhances throughput, making it suitable for commercial laboratories. Furthermore, the method’s validated parameters, including precision, accuracy, and linearity, meet regulatory requirements for analytical method validation, making it applicable for routine testing in pharmaceutical and cosmetic industries.
The validated method has certain limitations that warrant future consideration. Firstly, the analyte range was restricted to a specific concentration (0.8-6.4 μg/band), and extending this range could enhance its versatility for diverse applications. Secondly, organic solvents such as toluene and ethyl acetate raise environmental concerns, making exploring greener alternatives for a more sustainable approach essential. Additionally, the method was primarily validated for clove oil, and its applicability to other eugenol-containing matrices, such as pharmaceuticals or food samples, remains unexplored. Further validation across different matrices would improve its utility. Moreover, while HPTLC is a cost-effective technique, it may lack the sensitivity of more advanced methods like UPLC or LC-MS for detecting trace impurities. Integrating HPTLC with complementary techniques could enhance impurity profiling accuracy. Addressing these limitations through further studies and advancements would expand the method’s scope and improve its reliability for a wide range of analytical applications.
Conclusion
In this investigation, hydrodistillation was used to extract clove oil from Syzygium aromaticum L., and column chromatography was used to isolate the oil. The collected eugenol was characterized using TLC, FT-IR, ¹H NMR, and GC-MS, confirming a yield of 78%. A simple, precise, and validated HPTLC method was successfully developed to quantify eugenol extracted from S. aromaticum L. The technique demonstrated excellent specificity, linearity, accuracy, and precision, meeting regulatory standards for analytical validation. It proved robust against minor variations in mobile phase composition and detection wavelength, ensuring reliability for routine quality control. The method’s scalability allows for high-throughput analysis, making it suitable for pharmaceutical, cosmetic, and essential oil industries.
Conflict of interest
Nil
Acknowledgement
The authors would like to thank the principal of the Government College of Pharmacy and the principal scientific officer of the Drugs Testing Laboratory, Drugs Control Department, Bengaluru for providing the facilities to carry out the present research work. The authors also would like to express their gratitude to M/S Poornayu Research Labs in Bengaluru for providing gift sample of eugenol standard and spectra’s.
Supporting File
References
1. Khalil AA, Rahman UU, Khan MR, et al. Essential oil eugenol: sources, extraction techniques and nutraceutical perspectives. RSC Adv 2017;7(52):32669-81.
2. Haro-González JN, Castillo-Herrera GA, Martí-nez-Velázquez M, et al. Clove essential oil (Syzygium aromaticum L. myrtaceae): Extraction, chemical composition, food applications, and essential bioactivity for human health. Molecules 2021;26(21):6387.
3. Trease and Evans. Text book of Pharmacognosy. 15th edition. Elsevier Ltd; 2005:276.
4. Liu Y, Zhang G, Ma Y, et al. Eugenol inhibits NEAT1 as a ceRNA in pre-cancerous breast lesions. Heliyon 2025;11(1):e41353.
5. Yoon YE, Jung YJ, Lee S-J. The Anticancer Activities of Natural Terpenoids That Inhibit Both Melanoma and Non-Melanoma Skin Cancers. Int J Mol Sci 2024;25(8): 4423.
6. Bezerra DP, Militao GCG, Morais MCD, et al. The Dual Antioxidant/Prooxidant Effect of Eugenol and Its Action in Cancer Development and Treatment. Review. Nutrients 2017;9(12):1367.
7. Al-Sharif I, Remmal A, Aboussekhra A. Eugenol triggers apoptosis in breast cancer cells through E2F1/survivin down-regulation. BMC Cancer 2013;13:600.
8. Haro-González JN, Barbosa-Nuñez JA, Castillo-Herrera GA, et al. Clove essential oil and its major component, eugenol: a comparative study of their in vitro antioxidant and anticancer properties. Nat Prod Res 2024;1-8.
9. Damasceno ROS, Pinheiro JLS, Rodrigues, et al., Anti-Inflammatory and Antioxidant Activities of Eugenol: An Update. Pharmaceuticals 2024;17:1505.
10. Kanishk AK, Bhagirath VB. Syzygium aromaticum: A Useful Precious Spice. Indian Farmer 2025;12(1):17-27.
11. Al-Tawalbeh DM, Alawneh JM, Momani W, et al. Comparative antibacterial activity of clove extract against Pseudomonas aeruginosa. BMC Complement Med Ther 2025;25(1):7.
12. Salem M Z, Abo-Elgat W A A, Mansour M M A, et al. Antifungal activity of the monoterpenes carvacrol, p-cymene, eugenol, and iso-eugenol when applied to wood against Aspergillus flavus, Aspergillus niger, and Fusarium culmorum. BioResources 2025;20(1):393-412.
13. Tan Q, Pu J, Zhang M, et al. Eugenol targets laccase Cglac4 to subvert the pathogenicity of Colletotrichum gloeosporioides and control the postarvest fruit anthracnose. Postharvest Biol Technol 2025;221:113319.
14. Abdou A, Ennaji H, Maaghloud FE, et al. In silico and in vivo anti-inflammatory effect of eugenol and acetyleugenol. Sci Afr 2024;24:e02205.
15. Barb vioza JN, da Silva Maia Bezerra Filho C, et al. An overview on the anti‐inflammatory potential and antioxidant profile of eugenol. Oxid Med Cell Longev 2018;2018(1):3957262.
16. Da Silva FFM, Monte FJQ, de Lemos TLG, et al. Eugenol derivatives: synthesis, characterization, and evaluation of antibacterial and antioxidant activities. Chem Cent J 2018;12(1):34.
17. Alam MM. Synthesis and anticancer activity of novel Eugenol derivatives against breast cancer cells. Nat Prod Res 2023;37(10):1632-40.
18. Gopu CL, Aher S, Mehta H, et al. Simultaneous determination of cinnamaldehyde, eugenol and piperine by HPTLC densitometric method. Phytochem Anal 2008;19(2):116-21.
19. Mamatha M, Chaluvaraju KC, Shantharam U, et al. A study on isolation and purification of eugenol from clove by column chromatography. EJPMR 2018;5(5):383-6.
20. Rahimi AA, Ashnagar A, Nikoei Hamideh. Isolation and characterization of 4-allyl-2- methoxyphenol (eugenol) from clove buds marketed in Tehran city of Iran. Int J Chemtech Res 2012;4(1):105-8.
21. Foudah AI, Shakeel F, Alqarni MH, et al. Simultaneous Estimation of Cinnamaldehyde and Eugenol in Essential Oils and Traditional and Ultrasound-Assisted Extracts of Different Species of Cinnamon Using a Sustainable/ Green HPTLC Technique. Molecules 2021;26(7):2054.
22. Jain V, Sahu U, Chauhan NS, et al. HPTLC method development and quantification of marker compound eugenol in ayurvedic polyherbal formulations: Avipattikar churna. Tradit Med Mod Med 2023;06:9-16.
23. ICH. Validation of analytical procedures: text and methodology Q2(R1). [Internet]. 1996 [cited 2024 April 5]. Available from: https://database.ich.org/sites/default/files/Q2_R1__Guideline.pdf#:~:text=This%20document%20presents%20a%20discussion%20of%20the%20characteristics,applications%20submitted%20within%20the%20EC%2C%20Japan%20and%20USA..
24. Munishama Gowda YN, Chaluvaraju KC, Setty SR. A validated comparative study of RP-HPLC, GC-FID and UV spectrophotometric methods for the quantification of eugenol isolated from Syzygium Aromaticum L. J Pharmacogn Phytochem 2024;13(5):544-50.
25. Ahmad M, Kaur J, Khan S, et al. Optimization of a validated liquid chromatographic method with chemometric approach for estimation of eugenol from seed powder extract and perform antioxidant activity. Current Spectroscopy and Chromatography. 2024;10:e290324228475.
26. Ahmad W. Box-Behenken-Supported Develop-ment and Validation of UPLC Method for the Estimation of Eugenol in Syzygium aromaticum, Cinnamomum tamala, and Myristica fragrance. Separations 2023;10:248.