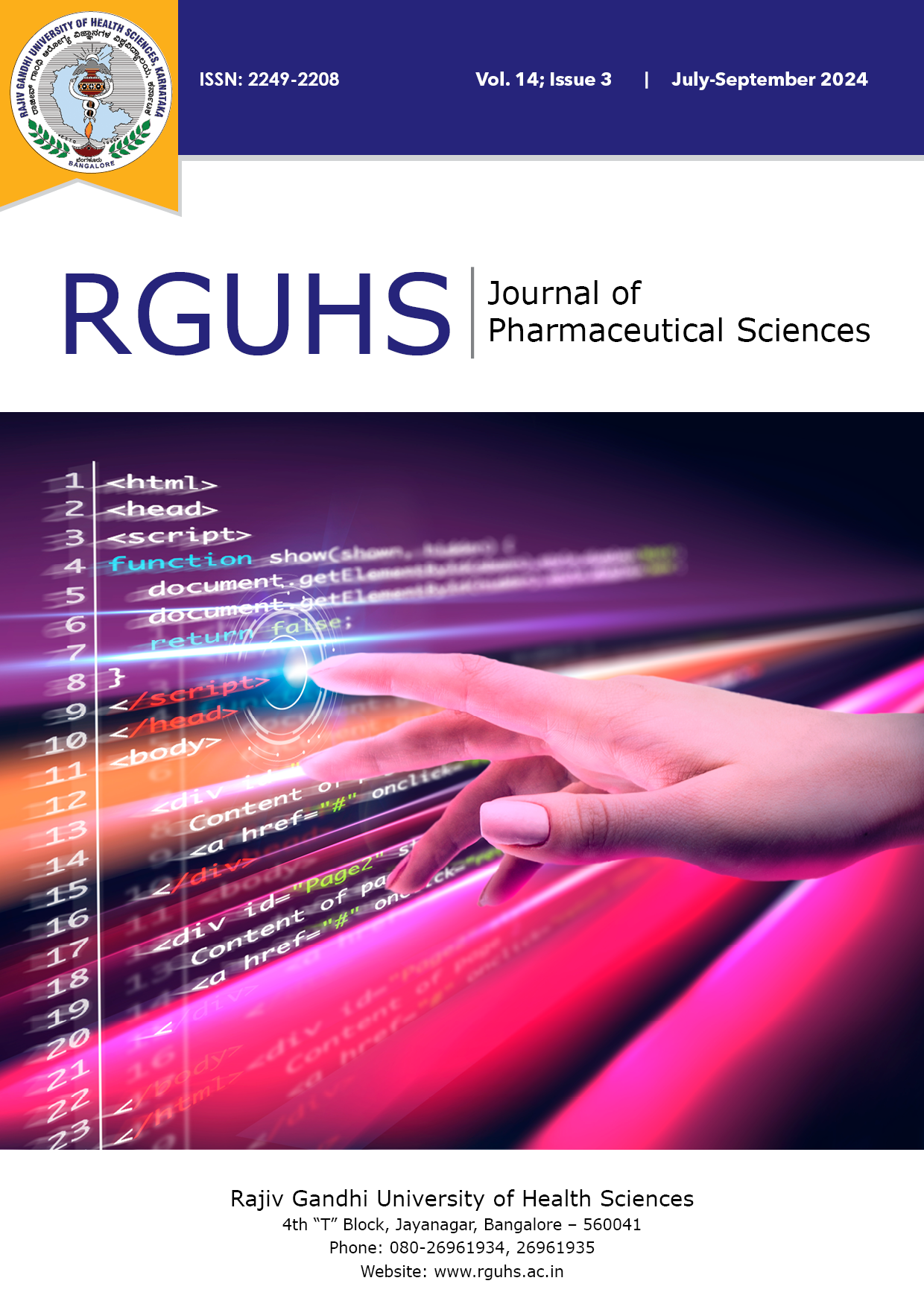
RJPS Vol No: 14 Issue No: 3 eISSN: pISSN:2249-2208
Dear Authors,
We invite you to watch this comprehensive video guide on the process of submitting your article online. This video will provide you with step-by-step instructions to ensure a smooth and successful submission.
Thank you for your attention and cooperation.
1Dr. Ganesh R Godge, Associate Professor, Department of Pharmaceutics, Dr. Vithalrao Vikhe Patil Foundation's College of Pharmacy, Vilad Ghat, M.I.D.C, Ahmednagar, Maharashtra, India.
2Dr. Vithalrao Vikhe Patil Foundation's College of Pharmacy, Ahmednagar, Maharashtra, India
3Dr. Vithalrao Vikhe Patil Foundation's College of Pharmacy, Ahmednagar, Maharashtra, India
4Dr. Vithalrao Vikhe Patil Foundation's College of Pharmacy, Ahmednagar, Maharashtra, India
5Dr. Vithalrao Vikhe Patil Foundation's College of Pharmacy, Ahmednagar, Maharashtra, India
6PRES’s Pravara Rural College of Pharmacy (Diploma), Pravaranagar, Loni, Maharashtra, India.
*Corresponding Author:
Dr. Ganesh R Godge, Associate Professor, Department of Pharmaceutics, Dr. Vithalrao Vikhe Patil Foundation's College of Pharmacy, Vilad Ghat, M.I.D.C, Ahmednagar, Maharashtra, India., Email: grgodge@yahoo.com
Abstract
The scientific community has been interested in nasal drug delivery as an additional method for administering vaccines and biomolecules, including proteins, peptides, and non-peptide medications. This interest arises from the fact that unstable drugs often undergo significant breakdown in the gastrointestinal tract (GIT) or experience first-pass hepatic metabolism when taken orally. The nasal route, on the other hand, provides a convenient alternative for delivering these drugs. The nasal route offers a parenteral drug delivery option which is advantageous for long-term therapy. Due to its high vascularization and permeability, the nasal mucosa promotes rapid absorption and onset of action. It is non-invasive, often utilized for both local and systemic therapy, and allows medication to enter the systemic circulation without any intermediate steps. Additionally, this approach is employed to circumvent the blood-brain barrier (BBB). While absorption promoters can enhance the absorption of large molecules like protein, nucleic acid, etc., nasal medication delivery provides higher absorption rates for low molecular weight pharmaceuticals. This article provides a comprehensive overview of current developments in intranasal drug delivery, covering various aspects, including the components involved, strategies for enhancing bioavailability, analysis of nasal drug formulation, and factors influencing nasal absorption.
Keywords
Downloads
-
1FullTextPDF
Article
Introduction
Nasal drug delivery has garnered significant attention recently due to its practical, promising, and reliable approach to systemic administration for medications. This method is particularly valuable for medications that cannot be administered orally due to their extensive metabolism by the liver's first-pass effect or within the gastrointestinal tract (GIT).1-6 Even parenteral methods can be inconvenient for long-term therapy. Consequently, several alternative approaches have been explored, and nasal drug delivery has proven to be effective. The nasal route offers several advantages, including high blood flow, bypassing of first-pass metabolism, a large surface area, a porous endothelium membrane, and easy accessibility. Moreover, the nasal mucosa exhibits greater medication permeability compared to the gastrointestinal tract (GIT) due to the absence of interference from pancreatic, gastric, and GI contents.7-14 Recent developments have expanded the spectrum of targeted body parts for nasal medication delivery, encompassing both local and systemic effects. This article provides an overview of the nasal drug delivery method.
Due to its huge surface area and elevated blood flow, which can be attained through self-administration, the nasal cavity plays a crucial role in the body for quicker and higher medication absorption. Medications given through the nasal cavity can vary in size ranging from microscopic micromolecules to massive macromolecules including protein/peptides, hormones, and vaccinations.15-17 According to reports, lipophilic medications can be effectively absorbed from the nasal cavity with a pharmacokinetic profile frequently equal to that of intravenous injections, and in many instances, their bioavailability can reach up to 100%. Fast absorption is facilitated by the surface area's size and high vascularization.18-19 The nasal route can be utilized as a backup parenteral administration route in an emergency. After entering the nasal cavity, drugs are quickly absorbed.
Anatomy and Physiology of Nasal Cavity
The nasal cavity refers to the interior of the nose, consists of two halves separated by the nasal septum. The nasal vestibule runs from the nasal entry to the nasopharynx. It is the front portion of the nasal cavity and is visible to the face through the nostril. Three central parts make up the nasal cavity.20-43
1. Respiratory region
2. Olfactory region
3. Nasal vestibule
The nasal cavity's lateral walls, including the folded structure, can increase the surface area of the nose by around 150 cm, giving it a remarkably large surface area compared to its modest volume. A conchae, also known as a turbinate, is a folded structure made up of the superior, inferior, and median turbinates. These structures enable the nose perform its main activities; the middle nasal airway is typically 1-3 mm broad.44-47
Mucous membrane covers the nasal cavity, which is separated into olfactory and non-olfactory epithelial sections. The nasal vestibule is a part of the non-olfactory epithelium and is lined with stratified squamous epithelial nasal cells, which resemble skin. Numerous microvilli, which are seen on the typical airway epithelium in the respiratory area are very important for the sizeable surface area that is available for drug absorption and transport. The nasal cavity's front and posterior halves are where the mucus layer is ejected. The mucus membrane that covers the nasal turbinate and the atrium contains goblet cells. It secretes mucus in the form of granules, which swell in the nasal fluid and add to the liquid mucus layer.48-53
A mucous membrane covers the nasal cavity. Water makes up 95% of mucus secretion, followed by mucin (2%), salt (1%), lipids (1%), and other proteins like albumin, lysozyme, and lactoferrin.
Mechanism of Drug Absorption
In case of nasal drug administration, passing through mucus is the initial stage in the drug's absorption from the nasal cavity. It is difficult for the charged and big medication molecules to cross. Two systems work together to absorb the medicine in the nasal drug delivery method.54-55
a. Paracellular absorption
The medication is transported through the epithelial cells via the aqueous route. In comparison to going the other way, the paracellular route is slower. The nasal epithelium's permeability through these channels is equivalent to that of the intestine. Intranasal absorption and the molecular weight of the water-soluble substances are inversely correlated with one another. For a combination with a molecular weight greater than 1000 Daltons, the paracellular route demonstrated low bioavailability.
b. Transcellular absorption
It involves transfer through a lipoidal channel and is known as the transcellular process. Its pace varies depending on how lipophilic the additional medications are, and it is responsible for transferring lipophilic medications. Active transport is used to traverse medicines, with cell membranes using a carrier to convey information across the tight junction opening.
Barriers to Nasal Absorption
Nasal medicine delivery systems are regarded as a lucrative choice for formulation scientists; it offers approachable formulation processes. Clinical efficacy of drugs delivered intra-nasally and toxicity are two factors that can differ. Here are a few things that prevent people from ingesting drugs through their noses.56-59
a. Low bioavailability
Compared to polar drugs, lipophilic pharmaceuticals are often better absorbed from the nasal cavity. With a near 100% bioavailability, the pharmacokinetic properties of lipophilic medicines are usually the same as those discovered after an intravenous infusion. The tmax for intravenous and oral antibiotics is extremely well illustrated by the nasal delivery of antibiotics. Drugs may enter epithelial cells through the tight junctions between cells, the receptor-mediated or vesicular transport pathways, or the transcellular route that takes advantage of concentration gradients. Polar drugs with molecular weights under 1000 Da can typically permeate the membrane through the latter mechanism. Larger peptides and proteins that use an endocytotic transport pathway can pass through the nasal membrane, but only in very minute amounts.60-64
b. Low membrane transport
The given formulation is often swiftly evacuated from the nasal cavity as a result of the mucociliary clearance process, which is another crucial step in restricted membrane transport. It is the case, especially for medicines that are challenging to absorb via the nasal membrane. For non-mucoadhesive liquid and powder formulations, the half-life of clearance is between 15 and 20 minutes, according to study. Additionally, it has been claimed that the residue of a formulation in the anterior portion of the nasal cavity can reduce clearance and increase absorption, as opposed to deposition further back in the nasal cavity. Contrary to nasal drops, which deliver the formulation throughout the entire nasal cavity, most nasal sprays from various brands have only been found to do so.
c. Enzymatic degradation
Another (usually less important) explanation for the limited transport of specific peptides and proteins through the nasal membrane is the potential for enzymatic destruction of the molecule, either within the lumen of the nasal cavity or during the passage across the epithelial barrier. Both sites have exopeptidases like mono- and di-aminopeptidases that can break peptides at their N and C termini as well as endopeptidases like serine and cysteine that can target interior peptide bonds. Enzyme inhibitors and saturating enzymes are strategies for overcoming this obstacle.
Factors Affecting Nasal Drug Absorption
1. Physicochemical properties of the drug
• Solubility
• Molecular weight
• Size
• Lipophilicity
• pka and partition coefficient
• Polymorphism
• Chemical properties
• Physical state
2. Biological factors
• Anatomy of the nasal cavity
• Biochemical feature
3. Physiological factors
• Nasal secretion
• Blood supply and neuronal regulation
• Mucociliary clearance
• Pathological condition
• Membrane permeability
• Environmental factor
4. Physicochemical properties of formulation
• Osmolarity
• Viscosity
• pH
• the physical form of formulation
Strategies to Improve Nasal Drug Absorption
Numerous obstructions in the nasal canal prevent some drugs from being absorbed. Some methods have been tried with success to boost the absorption of nasal medications.
Numerous medications with a high water solubility have a low transepithelial permeability and possibly a low bioavailability. In order to increase their bioavailability and permeability, penetration enhancers are frequently utilized. The structural alterations brought on by permeation enhancers can be reversed. It is generally acknowledged that these substances increase the permeability of the epithelial cell layer by altering the phospholipid bilayer, while the specific mechanism for improving medicine absorption and penetration is unknown.
a. Nasal enzyme inhibitors
To reduce the activity of the enzymes present in the nasal cavity, many types of enzyme inhibitors, including protease and peptidase inhibitors, are utilized in the formulation of nasal medication delivery preparations.
b. Structural modification
The chemical structure of active medication molecules can be changed without affecting their pharmacological activity in order to increase nasal absorption.
c. Permeation enhancer
To increase nasal absorption, various kinds of permeation enhancers, including surfactants, fatty acids, phospholipids, cyclodextrins, bile salts, etc. have been studied.
d. Particulate drug delivery
Drugs are occasionally included in carriers that increase the retention capacity of the nasal cavity and guard against drug exposure to the environment. A few different kinds of pages that can be used are microspheres, liposomes, nanoparticles, and niosomes.
e. Prodrug approach
Sometimes, nasal formulation prodrugs are mainly utilized to promote stability and solubility. Prodrug is an inactive chemical component that becomes active when it reaches the target location.
f. Bioadhesive polymer
Bioadhesive polymers boost a drug's nasal absorption and residence time by exerting an adhesive force between the formulation and nasal mucosa. This lengthens a drug's time in the nasal cavity and reduces mucociliary clearance of the formulation.
g. In situ gel
When injected into the nasal cavity, these formulations become a gel when exposed to stimuli including temperature, pH, and ionic concentration. A gel's thick nature prevents ciliate motility from rapidly draining the formulation.
Excipients Used in Nasal Formulations65-69
Nasal formulations use a variety of excipient types. The following excipients are frequently used and added.
1. Bioadhesive polymers
A substance known as a bioadhesive polymer can interact with biological material through interfacial forces and adhere to that material for long periods of time. They are also known as mucoadhesive if the natural substance is a mucus membrane. The process of mucoadhesion can be described in terms of attractive molecular interactions, which include forces like Van der Waals, electrostatic interactions, hydrogen bonds, and hydrophobic interactions. The bioadhesive ability of a polymer is influenced by its chemical make-up, environmental pH, swelling, and physiological factors.
2. Gelling agent
Gelling agents thicken or make the formulation more viscous, lengthen nasal retention, and stop spillage from the nasal cavity as a result of gravity and mucociliary clearance. Nasal administration typically makes use of polymers such as poloxamer, chitosan, ethylcellulose, pectin, xylulose, Carbopol (a pH-responsive polymer), guar gum (an ion-responsive polymer), and others. Such polymers show stimulus-responsive sol-gel transition when exposed to various physiological stimuli, including temperature, pH, ionic concentration, exposure to a particular enzyme, and so on. The installation of nasal formulation (in sol form) is made easier by the unique property of sol-gel evolution. When exposed to the physiological environment, it transforms into a gel with the proper viscosity, enhancing nasal retention.
3. Penetration enhancers
In nasal drug delivery, chemical penetration enhancers are commonly utilised. The following is a classification of chemical penetration enhancers:
a. Solvents
b. Alkyl methyl sulphoxides
c. Pyrrolidones
d. 1- Dodecylazacycloheptan-2-one
e. Surfactants
f. Buffers
Nasal formulations are often administered in small doses of 25 to 200 μL, with 100 μL being the most typical dosing volume. Therefore, nasal secretions may alter the pH of the dose that is provided, which may modify the amount of unionized drug that is available for absorption. Therefore, to keep the pH in place, a significant formulation buffer capacity may be needed.
5. Solubilizers
Nasal medication administration in solution is always constrained by the drug's aqueous solubility. Glycols, small amounts of alcohol, Transcutol (diethylene glycol monoethyl ether), medium chain glycerides, and Labrasol (saturated polyglycolide C8-C10 glyceride) are examples of conventional or co-solvents that can be used to boost the solubility of medications. Other chemicals can be used, such as cyclodextrins or surfactants combined with lipophilic absorption boosters, such as HP-ß-Cyclodextrin, a biocompatible solubilizer and stabiliser. Their impact on nasal irritancy should be taken into account in these circumstances.
6. Preservative
The most popular nasal formulations are aqueous, which need preservatives to prevent bacteria development. In nasal formulations, preservatives such as benzalkonium chloride, phenyl ethyl alcohol, parabens, EDTA, and benzyl alcohol are widely utilized.
7. Antioxidants
Antioxidants could be required in very small amounts to prevent medication oxidation. Antioxidants, such as sodium bisulfite, sodium metabisulfite, butylated hydroxytoluene, and tocopherol, are often used. Antioxidants frequently do not cause nose irritation or interfere with how medications are absorbed. The formulation development program should take into account how antioxidants and preservatives interact chemically and physically with drugs, excipients, manufacturing procedures, and packaging components.
8. Humectants
Mucous membranes may become dry and crust over in cases of chronic and allergic diseases. Additionally, it has been demonstrated that a number of antioxidants and preservatives might irritate the nasal passages, particularly when used in higher dosages. Therefore, humectants can be added, especially to gel-based nasal treatments. While preventing nose irritation, humectants have little effect on how well pills are absorbed. Common examples are mannitol, sorbitol, and glycerine.
9. Surfactants
The addition of surfactants to nasal dosage forms can alter the permeability of nasal membranes, potentially enhancing nasal drug absorption.
Formulations of Nasal Drug Delivery70-74
1. Liquid dosage forms
Nasal drops: Among all formulations, nasal drops are one of the simplest and most efficient administration systems. The requirement for precise dosing is this system's biggest flaw.
Nasal emulsion: In comparison to other liquid nasal delivery methods, intranasal emulsions have received less study attention. Nasal emulsions are advantageous for local application due to their viscosity.
Nasal spray: Formulations for suspensions and solutions can be used to make nasal sprays. Due to the accessibility of metered dose pumps and actuators, a nasal spray can deliver a precise dose anywhere between 25 and 200 μL.
2. Semisolid dosage forms
These kinds of nasal drug delivery systems are frequently created employing semisolid materials, like gels, ointments, and liquids with polymers that gel at particular pH levels.
Nasal gels: Viscous, thickened liquids or suspensions are nasal gels. Nasal gel has several advantages, including a reduction of anterior formulation leakage, a reduction in flavor effect, and a decrease in post-nasal dripping due to its high viscosity.
3. Solid dosage forms
The use of solid dosage forms for intranasal drug delivery is growing in popularity. These formulations can cover the vasculature within the epithelium of nasal mucosa, making them better suited for pulmonary medication delivery and similar purposes.
Nasal powders: If solution and suspension dosage forms cannot be produced, generally due to insufficient drug stability, powder dosage forms may be devised. The lack of a preservative and the superior potency of the medication in the formulation are the advantages of a nasal powder dosage form. However, the solubility, particle size, aerodynamic properties, and nasal irritancy of the active ingredient and excipients determine if the powder formulation is appropriate.
4. Novel drug formulations
The development of liposome, microsphere, and nanoparticle-based nasal formulations for intranasal drug administration has been supported by a number of factors. To improve stability, membrane penetration, and retention time in the nasal cavity, these systems may also contain enzyme inhibitors, nasal absorption boosters, or mucoadhesive polymers in addition to the medication.
Nanoparticles: Nanoparticles are solid colloidal particles with sizes ranging from 1 to 1000 nm. They are macromolecules that have potential therapeutic uses as adjuvants in vaccines or as drug carriers where the active ingredient is dissolved, entrapped, encapsulated, adsorbed, or chemically bound. Nanoparticles may have a number of benefits due to their small size. Nevertheless, the smallest nanoparticles may completely penetrate the mucosal membrane by the paracellular pathway because tight junctions only have a diameter of 3.9 to 8.4.
Solid lipid nanoparticles and nanostructured lipid carriers: SLNs are first-generation lipid nanocarriers that offer physical stability, targeted drug delivery, and labile pharmaceuticals that are susceptible to environmental changes. They can also contain a variety of treatments, including DNA, small interfering RNA (siRNA), polysaccharides, vaccine antigens, and big biomacromolecules.
A layer of surfactants surrounds the solid lipid matrix (triglycerides, waxes, sterols, and fatty acids) that makes up SLNs, which are typically spherical with a diameter of 50–1000 nm. Their stability may be primarily influenced by the kind and quantity of lipids used.
Polymeric nanoparticles: Colloidal particles with a size range of 1 to 1000 nm are known as polymeric nanoparticles. They are capable of carrying both hydrophobic and hydrophilic substances, including proteins, small molecules, biological macromolecules, and vaccines, inside or on the surface of their polymeric core. Additionally, they can quickly alter their surfaces to target tissue.
These nanoparticles can be produced more accurately using either synthetic or natural materials. Polymeric nanoparticles are produced by nanoprecipitation, emulsification, ionotropic gelation, and microfluidic methods. Greater control of the loading rate and release kinetics is possible by altering composition, stability, surface loading, and responsiveness.
Inorganic nanoparticles: Inorganic nanoparticles are potential agents for a variety of drug delivery applications due to their favorable physicochemical properties, including controlled release, biocompatibility, stability, and huge surface area. They can be meticulously formulated and designed such that they can have a variety of shapes, sizes, and geometries. However, their usage in therapeutic settings is constrained by their low solubility and probable toxicity. By modifying the surfaces of inorganic nanoparticles to improve their efficiency for drug delivery, these limitations can be circumvented.
Liposomes: Liposomes, which are phospholipid vesicles with lipid bilayers enclosing one or more water compartments, may contain drugs and other substances. The effective encapsulation of small and large molecules with varied hydrophilicity and pKa values is one advantage of liposomal drug delivery methods. They have been found to enhance nasal absorption of peptides like insulin and calcitonin via improving membrane penetration. Investigation and comparison of the permeability of insulin solution with or without pre-treatment with sodium glycocholate (GC) to insulin-entrapped liposomes through rabbit nasal mucosa was carried out. When the liposome suspension and insulin solution were compared, the liposome that had received GC pre-treatment permeated more effectively.
Microsphere: The use of microsphere technology in the development of formulations for nasal medicine administration has been considerable. Microspheres are frequently based on mucoadhesive polymers like chitosan, alginate, etc. for intranasal drug administration. Additionally, microspheres may prevent the drug from being metabolized by enzymes and maintain drug release, lengthening the duration of the effect.
Evaluation of Nasal Drug Formulations
Ex vivo, in vitro, and in vivo models are extensively used in nasal drug delivery investigations. Numerous investigations can employ the different models. Permeation and diffusion studies can be performed using in vitro methods, and pharmacokinetic profiles of drugs can be determined using in vivo models. Finally, nasal perfusion can be investigated using an ex vivo method. Below, we shall discuss more details about these models.75-78
In vitro studies
In vitro research can be used to study and regulate the mechanical elements of nasal medication absorption and transport. The most important nasal medication absorption and permeability testing involves in vivo research. The main cell types used to test nasal permeability and absorption are RPMI 2650 and CaCo-2. Note that while these cellular models provide insight into transport across cells or paracellular structures, concurrent factors like mucus, mucins, clearance, and anatomical and physiological components involved in maintaining the nose's functionality may also have an effect on the absorption. Furthermore, the essential transport from the mucosa to the receiving neurons is not accurately represented by the receiving lumen in the cellular models.
a) RPMI 2650, a cell culture model of the nasal barrier
The human nasal septum squamous cell carcinoma and human nasal epithelial tissue used to create RPMI 2650 are both naturally occurring tumors. The human RPMI 2650 cell line is frequently utilized for investigations on nasal metabolism and toxicity tests since it never forms monolayers and only develops into multilayers. The RPMI 2650 cell line has been employed in drug permeation experiments even though it is not appropriate for drug transport studies. Confluent cell monolayers are formed by serially passaged RPMI 2650 cells under controlled circumstances. The medium used for cell culture and cellular differentiation supplements are among these conditions. Transepithelial electrical resistance (TEER), a property of tight connections formed by each monolayer facilitates medication transfer.
b) CaCo-2 cell line
The CaCo-2 cell line, which has been in use for three decades and is appropriate for assessing formulation nasal absorption, is another common cell culture model. The cell line, which slowly divides into several monolayers, is obtained from human colon cancer. The most accurate model for assessing drug absorption and permeability across the intestinal epithelium is Caco-2 cell culture.
In vivo nasal absorption studies
In vivo model
• Animal models for nasal absorption studies
Studies on the nasal absorption of medications from their formulation can be conducted using animals of two different types: whole animals, also known as in vivo models, and isolated organ perfusion models, also known as ex vivo models. Nasal drug delivery models can be utilized for pharmacokinetic and pharmacodynamic studies, toxicological and electrophysiological investigations, and evaluations of drug transporter interactions and the nasal barrier in addition to detecting and testing nasal drug absorption and permeation.
For the investigation of nasal administration methods, efficient in vivo models are essential. Understanding the architecture of the animal's nasal cavity is crucial before selecting the best animal model for in vivo nasal absorption research. The initial animal model was the rat, which was employed in investigations on nasal absorption starting in the late 1970s. Later, mouse, rabbit, dog, sheep, and monkey were also used. Table 3 presents representative reports on numerous experimental species and strains used in studies on nasal medication administration.
Rat and mouse models are useful for preliminary studies on medication absorption from the nose to the brain. However, the rabbit, dog, and sheep models are more frequently used in pharmacokinetic research.
Ex vivo model
For the development of drugs for nasal delivery, reliable study models are essential. In ex vivo research, the nasal mucosa of experimental or killed animals is typically employed to assess the toxicity of excipients and the transmucosal transport of medicines. Ex vivo excised animal tissue models from rats, rabbits, dogs, sheep, primates, and even humans are frequently employed in study.
Tissues that have been removed can be used to study toxicity, efflux, metabolism, and penetration. Ex vivo models for medication delivery through the nose have certain drawbacks despite their many benefits. The thickness of animal nasal epithelial tissues and the absence of interstitial flow rate underneath the mucosa are the two most important limiting factors. It becomes challenging to translate permeability experiment findings to in vivo models. When using nasal drug administration, it is crucial to look into various aspects that could impact the degree and rate of drug absorption. These elements include medication formulation, permeation processes, and metabolic stability.
The most popular ex vivo nasal perfusion model for drug permeability is the Ussing chamber. This model's uncomplicated implementation makes it easy to keep track of and maintain tissue viability throughout the investigation. Research on permeability can be used to pinpoint and describe (compound) specific carrier-mediated transport pathways as well as measure passive diffusion, active transport, and efflux transport. In these animals, the efflux pumps found in the nasal mucosa must be investigated both with and without substances that might inhibit these pumps. Additionally, the Ussing chamber model enables comparisons between medication delivery in the olfactory and nasal respiratory mucosa.
In conclusion, ex vivo models are widely used for drug screening during the early stages of drug development, especially for research examining drug distribution.
Conclusion
One of the most promising and adaptable delivery methods for medication during the past ten years has been through the nasal cavity. Its unique ability to carry drugs to the brain without passing via the liver's first-pass metabolism holds special promise for drug delivery. A growing body of research on nasal drug administration suggests that it may be used for treatments that are challenging to manufacture and administer. They examined the potential for using different pharmacological dosage forms for local or systemic medication administration. It stands to reason that this review will contribute to a better knowledge of intranasal formulations and their future development to meet specific therapeutic objectives. In order to realize its full potential, a number of technological and practical issues have been highlighted in this review.
Conflict of Interest
Nil
Acknowledgement
The authors wish to express their gratitude to all their mentors as well as Principal at Dr. V. V. P. Foundation's College of Pharmacy in Ahmednagar. The researchers whose works are cited and listed in this manuscript's references are acknowledged by the authors for their enormous assistance. The writers also acknowledge all authors, editors, and publishers of the books, journals, and articles that served as the foundation for this article's review and discussion.
Supporting File
References
- Arora P, Gary S. Permeability issues in nasal drug delivery. Drug Discov Today 2002;7:967-975.
- Asane GS, Nirmal SA, Rasal KB, Naik AA, Mahadik MS, Madhusudan Rao YM. Polymers for mucoadhesive drug delivery system: A current status. Drug Dev Ind Pharm 2008;34:1246-66.
- Marttin E, Nicolaas GM, Schipper J, Coos V, Frans WH. Nasal mucociliary clearance as a factor in nasal drug delivery. Adv Drug Del Rev 1997;29: 13-38.
- Choi HG, Jung JH, Ryu JM. Development of in situ-gelling and mucoadhesive acetaminophen liquid suppository Int J Pharm 1998;165:33-44.
- Graff LC, Pollock GM. Nasal drug administration: potential for targeted central nervous system delivery. J Pharm Sci 2005;94:1187-1195.
- Lipworth BJ, Jackson CM. Safety of inhaled and intranasal corticosteroids: lessons for the new millennium. Drug Saf 2000;23:11-33.
- Wiseman JW. Steroid hormone enhancement of gene delivery to a human airway epithelial cell line in vitro and mouse airway in vivo. Gene The 2001;8:1562-1571.
- Krishnamoorthy R, Mitra NK. Prodrugs for nasal delivery. Adv Drug Deliv Rev 1998;29:135-146.
- Duquesnoy C, Mamet JP, Sumner D, Fuseau E. Comparative clinical pharmacokinetics of single doses of sumatriptan following subcutaneous, oral, rectal and intranasal administration. Eur J Pharm Sci 1998;6:99-104.
- Singh AK. Nasal cavity: A promising transmucosal platform for drug delivery and research approach from nasal to brain targeting. J Drug Deliv Therapeut 2012;23:22-33.
- Chajed S, Sangle S, Barhate S. Advantageous nasal drug delivery system: a review. International Journal of Pharmaceutical Science and Research 2011;2(6):1322-1336.
- Behl CR, Pimplaskar NK, Sileno AP, Demeireles J, Romeo VD. Effect of physicochemical properties and other factors on nasal drug delivery. Adv Drug Del Rev 1998;29:89-116.
- Cauna N. Blood and nerve supply of the nasal lining. In: Proctor DF, Andersen IB (Eds.), Chapter in The Nose: Upper Airway Physiology and the Atmospheric Environment. Amsterdam: Elsevier Biomedical Press; 1982. p. 45-69.
- Illum L. Drug transport from the nasal cavity to the central nervous system. Eur J Pharm Sci 2000;11: 1-18.
- Parvathi M. Intranasal drug delivery to the brain: an overview. International Journal of Research in Pharmacy and Chemistry 2012;2(3):889-895.
- Sarkar MA. Drug metabolism in the nasal mucosa. Pharm Res 1992;9:1-9.
- Merkus FW, Verhoef JC, Schipper NG, Martin E. Nasal mucociliary clearance as a factor in nasal drug delivery. Adv Drug Deliv Rev 1998;29:13-38.
- Charlton S, Jones NS, Davis SS, Illum L. Distribution and clearance of bioadhesive formulations from the olfactory region in man: Effect of polymer type and nasal delivery device. Eur J Pharm Sci 2007;30: 295-302.
- Dondeti P, Zia H, Needham TE. Bioadhesive and formulation parameters affecting nasal absorption. Int J Pharm 1996;127:115-133.
- Dahl R, Mygind N. Anatomy, physiology and function of the nasal cavities in health and disease. Adv Drug Deliv Rev 1998;29:3-12.
- Machida M. Effects of surfactants and protease inhibitors on nasal absorption of recombinant human granulocyte colony-stimulating factor (rHGCSF) in rats. Biol Pharm Bull 1994;17:1375-1378.
- Watanabe H, Tsuru H. Nippon Yakurigaku Zasshi 1999;113:211-218.
- Cornaz AL, Buri P. Nasal mucosa as an absorption barrier. Eur J Pharm Biopharm 1994;40:261-270.
- Kumar GP, Kiran S. Strategies and prospects of nasal drug delivery systems. Indian J Pharm Sci Res 2012;2(1):33-41.
- Corbo DC. Characterization of the barrier properties of mucosaln membranes. J Pharm Sci 1990;79:202-206.
- Sakane T. The transport of a drug to the cerebrospinal fluid directly from the nasal cavity: the relation to the lipophilicity of the drug. Chem Pharm Bull (Tokyo) 1991;39:2456-2458.
- Ohwaki K, Ando H, Watanabe S, Miyake Y. Effects of Krenistsky, Amino acid ester prodrugs of acyclovir, Antiviral dose, pH, and osmolarity on nasal absorption of secretin. Chem Chemother 1992;3:157-164.
- Clement P, Roovers MH, Francillon C, Dodion P. Dose ranging, placebo-controlled study of cetirizine nasal spray in adults with perennial allergic rhinitis. Allergy 1994;49:668-672.
- Harshad P, Anand B, Dushyant S. Recent techniques in nasal drug delivery: A review. Int. J. Drug Dev. Res. 2010;2(3):565-72.
- Sahua BP, Sharma HK, Dasc MK. Development and evaluation of a mucoadhesive nasal gel of felodipine prepared with the mucoadhesive substance of Dillenia indica L. Asian J Pharm Sci 2011; 5:175-187.
- Kashid V, Nikam V, Gayakawad S, Mahale M. Nasal gel as promising mucosal drug delivery. World Journal of Pharmaceutical Research 2016;6: 891-912.
- Zaheer A, Swamy S. Mucoadhesive Polymers: Drug Carriers for Improved Nasal Drug Delivery. Indian Journal of Novel Drug Delivery 2012;4(1):2-16.
- Abdel-Bar HM, Abdel-Reheem AY, Awad GAS, Mortada ND. Evaluation of brain targeting and mucosal integrity of nasally administrated nanostructured carriers of a CNS active drug, clonazepam. J Pharm Pharm Sci 2013;16:456-69.
- Nagaraju R, Pallavi K, Deepthy K, Haritha M. Formulation and evaluation of niosomal nasal drug delivery system of folic acid for brain targeting. Curr Drug Discov Technol 2013;10(4):270-82.
- Alam MI, Baboota S, Ahuja A, Ali M, Ali J, Sahni JK. Intranasal infusion of nanostructured lipid carriers (NLC) containing CNS-acting drug and estimation in brain and blood. J Control Release 2013;20:247-51.
- Sharma S, Lohan S, Murthy RSR. Formulation and characterisation of intranasal mucoadhesive nanoparticulate and thermo-reversible gel of levodopa for brain delivery. Drug Dev Ind Pharm 2013;40:869-78.
- Boche M, Pokharkar V. Quetiapine nanoemulsion for intranasal drug delivery: evaluation of braintargeting efficiency. AAPS Pharm Sci Tech 2017;18:686-96.
- Tang S, Wang A, Yan X, Chu L, Yang X, Song Y, et al. Brain-targeted intranasal delivery of dopamine with borneol and lactoferrin co-modified nanoparticles for treating Parkinson’s disease. Drug Deliv 2019;26:700-7.
- Rathananand M, Kumar D, Shirwaikar A, Kumar R, Sampath Kumar D, Prasad R. Preparation of mucoadhesive microspheres for nasal delivery by spray drying. Ind J Pharm Sci 2007;69:651.
- Maitani Y, Asano S, Takahashi S, Nakagaki M, Nagai T. Permeability of insulin entrapped in liposome through the nasal mucosa of rabbits. Chem Pharm Bull 1992;40:1569-72.
- Stevens J, Suidgeest E, Van Der Graaf PH, Danhof M, De Lange ECM. A new minimal-stress freely-moving rat model for preclinical studies on intranasal administration of CNS drugs. Pharm Res 2009;26:1911-1917.
- Najafabadi AR, Moslemi P, Tajerzadeh H. Intranasal bioavailability of insulin from carbopol-based gel spray in rabbits. Drug Deliv J Deliv Target Ther Agents 2004;11:295-300.
- Sato H, Nave R, Nonaka T, Yoshihisa N, Atsuhiro N, Mochizuki T, et al. Uptake and metabolism of ciclesonide and retention of desisobutyrylciclesonide for up to 24 hours in rabbit nasal mucosa. BMC Pharmacol 2007;7:7.
- Karasulu E, Yavaşoǧlu A, Evrenşanal Z, Uyanikgil Y, Karasulu HY. Permeation studies and histological examination of sheep nasal mucosa following administration of different nasal formulations with or without absorption enhancers. Drug Deliv 2008;15:219-225.
- Moore SJ, Smith JD, Greenlee MHW, Nicholson EM, Richt JA, Greenlee JJ. Comparison of two US sheep scrapie isolates supports identification as separate strains. Vet Pathol 2016;53:1187-1196.
- Micieli F, Santangelo B, Napoleone G, Di Dona F, Mennonna G, Vesce G. Intranasal fentanyl for acute severe pain episodes control in a dog. Vet Anaesth Analg 2017;44:1400-1401.
- Saccone PA, Lindsey AM, Koeppe RA, Zelenock KA, Shao X, Sherman P, et al. Intranasal opioid administration in Rhesus monkeys: PET imaging and antinociception. J Pharmacol Exp Ther 2016;359:366-373.
- Shaikh S, Merekar AN, Godge GR, Gaikwad MR. Formulation and in-vitro evaluation of buccal mucoadhesive tablets of captopril by using natural and synthetic polymers. World Journal of Pharmaceutical Research 2016;5(7):1296-1315.
- Kute VC, Godge G. Preparation & in-vitro evaluation of inclusion complexes of simvastatin tablet with cyclodextrins. World Journal of Pharmaceutical Research 2016;5(2):1022-1041.
- Godge GR, Palwe VD, Pawar PY. Formulation development and in-vitro evaluation of sustained release tablets of telmisartan by solid dispersion technology. Asian Journal of Pharmaceutical Technology & Innovation 2016;4(17):131-139.
- Chemate SZ, Godge GR, Pawa KK, Rupnar KA. Preparation and evaluation of hollow calcium pectinate beads for floating-pulsatile drug delivery. Turk J Pharm Sci 2016;13(1):91-102.
- Raskar MA, Godge GR, Chitale AB, Giri PD. Validated simultaneous spectrophotometric estimation of telmisartan, hydrochlorothiazide and amlodipine besylate in combined tablet dosage form. Der Pharmacia Lettre 2015;7(11):120-124.
- Godge GR, Misal AV, Pawar PY. Formulation and evaluation of mouth dissolving tablet with taste masking resin. Int J Life Sci Rev 2015;1(7): 253-263.
- Godge G, Labade S, Misal A. Oral bioavailability improvement using solid dispersion techniques: a review. Int J Life Sci Rev 2015;1(7):243-252.
- Hiremath S, Godge G, Sonawale B, Shirsath R. Pharmaceutical advances in cyclodextrin inclusion complexes for improved bioavailability of poorly-soluble drugs. Int J Pharm Sci Nanotech 2015;8(3):2894-2905.
- Godge GR, Labade SP. Preparation of solid dispersion of poorly water soluble drug formulation and consideration. Int J pharm Sci Res 2015;6(5):897-903.
- Godge GR, Hiremath SN. An investigation into the characteristics of natural polysaccharide: polymer metoprolol succinate tablets for colonic drug delivery. Mahidol University Journal of Pharmaceutical Sciences 2014;41(2):7-21.
- Godge GR, Hiremath SN. Colon targeted drug delivery system: a review. Int J Pharm Drug Ana 2014;2(1):35-48.
- Godge GR, Hiremath SN. Development and evaluation of colon targeted drug delivery system using natural polysaccharides/polymers. Dhaka Univ J Pharm Sci 2014;13(1):105-113.
- Hiremath S, Godge G. Preparation and in vitro evaluation of inclusion complexes of nelfinavir with chemically modified β-cyclodextrins. Dhaka Univ J Pharm Sci 2012;11(2):107-116.
- Godge G, Hiremath S. Colonic delivery of film-coated meloxicam tablets using natural polysaccharide polymer mixture. Int Curr Pharm J 2012;1(9):264-271.
- Hiremath SN, Kharia AA, Godge GR. Formulation strategies for low absorption window antihypertensive agents. Res J Pharm Technol 2010;3(1):83-91.
- Hiremath SN, Godge GR. Recent advances in pharmaceutical approaches to colon specific drug delivery. Pharm Tech 2011;4:1-8.
- Kharia AA, Hiremath SN, Omray LK, Yadav R, Godge GR. Gastro-retentive Drug Delivery System. Indian Drugs 2011;48(5):7-15.
- Hiremath SN, Godge GR, Kharia AA, Vaidya VR. Studies on the preparation, characterisation and solubility of β-cyclodextrin-nelfinavir inclusion complexes. JPRHC 2010;2(3):279-284.
- Vaidya VR, Karodi RS, Mohite MT, Hiremath SN, Godge GR. Formulation optimisation of mucoadhesive buccal tablets of carvedilol using 32 full factorial designs. Deccan J Pharmaceutics Cosmetology 2010;1(2):7-20.
- Hiremath SN, Kharia AA, Godge GR. Formulation strategies for low absorption window antihypertensive agents. Res J Pharm Technol 2010;3(1):83-81.
- Sanap D, Garje M, Godge G, Probiotics, their health benefits and applications for development of human health: a review. J Drug Deliv Ther 2019; 9(4s): 631-640.
- Sanjay SD, Godge GR. Recent trends in medicated chewing gum technology: a review on history, formulation strategies and characterisation. Int J Pharm Sci Rev Res 2019;59(2):72-85.
- Hiremath SN, Godge GR, Kulkarni PS, Landge RB. Discussion on tablets with special emphasis on formulation and coating defects: A review. IJCRT 2021;9(7):124-139.
- Godge GR, Zarekar SB, Padale PJ. Nanosuspension technologies: A innovative approach in drug delivery of sparingly soluble drugs. IJCRT 2021;9(7): 409-420.
- Godge GR, Ghume VK, Bidave BB, Golhar AR. 3D printing technology in pharmaceutical drug delivery. Int J Pharm Sci Rev Res 2021;68(1): 15-20.
- Godge GR, Garje MA, Dode AB, Tarkase KN. Nanosuspension technology for delivery of poorly soluble drugs and its applications: a review. Int J Pharm Sci Nanotech 2020;4:4965-4978.
- Godge GR, Arjun GM, Balaprasad DA, Machindra DA, Vinayak WD, Jalindar FS. Validated spectrophotometric method for simultaneous estimation of fenofibrate and atorvastatin in synthetic mixture and in bulk tablet dosage form. WJPMR 2020;6(7):170-176.
- Landge RB, Kulkarni PS, Godge GR, Hiremath SN. A detailed report on tablets with special emphasis on its formulation and manufacturing technology. IJCRT 2022;10(3):f624-f641.
- Raskar MA, Kate PA, Mungse SS, Godge GR.J. Validated Simultaneous Derivative Spectrophotometric Estimation of Azithromycin, Fluconazole and Secnidazole in Bulk and Pharmaceutical Formulation. J drug deliv. ther 2023;13(11): 1-6.
- Raskar MA, Mungse SS, Kate PA, Godge GR. Validated Simultaneous Derivative Spectrophotometric Estimation of Diflunisal and Lignocaine in Bulk and Pharmaceutical Formulation. J drug deliv. ther. 2023; 13(8):51-55.
- Godge GR, Bharat SC, Shaikh AB, Randhawan BB, Raskar MA, Hiremath SN. Formulation Perspectives in Topical Antifungal Drug Therapy: A Review. J drug deliv. ther 2023; 13(5):110-119.