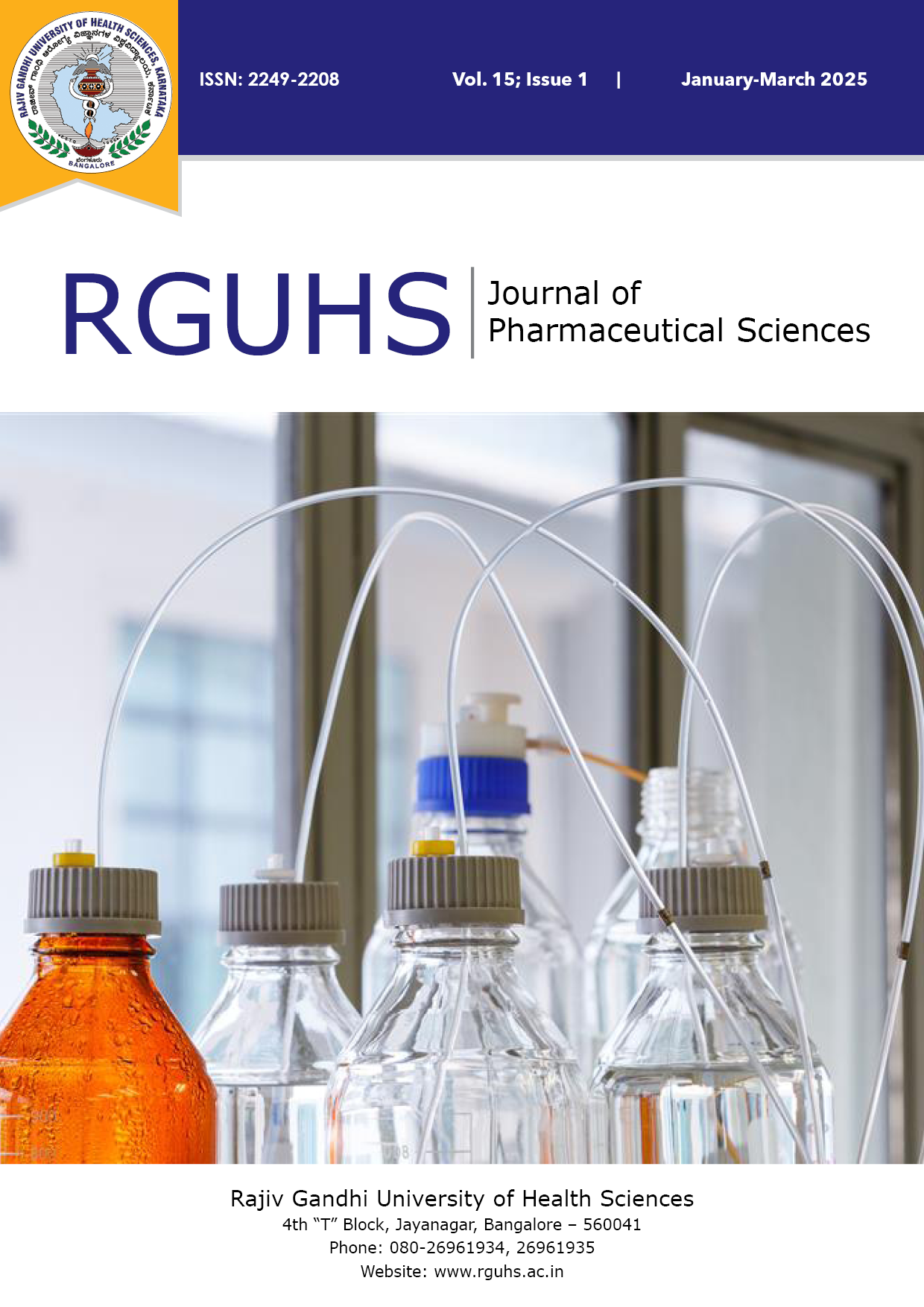
RJPS Vol No: 15 Issue No: 1 eISSN: pISSN:2249-2208
Dear Authors,
We invite you to watch this comprehensive video guide on the process of submitting your article online. This video will provide you with step-by-step instructions to ensure a smooth and successful submission.
Thank you for your attention and cooperation.
1Ms. K Pooja, Department of Pharmaceutics, HKES’s Matoshree Taradevi Rampure Institute of Pharmaceutical Sciences, Gulbarga, Karnataka, India.
2Department of Pharmaceutics, HKES’s Matoshree Taradevi Rampure Institute of Pharmaceutical Sciences, Gulbarga, Karnataka, India.
*Corresponding Author:
Ms. K Pooja, Department of Pharmaceutics, HKES’s Matoshree Taradevi Rampure Institute of Pharmaceutical Sciences, Gulbarga, Karnataka, India., Email: Poojank1992@gmail.com
Abstract
Due to a lack of effective treatment factor or drug delivery systems, many diseases go untreated, especially when side effects and toxicities are the main cause of concern. Consequently, we chose novel drug delivery system. The structure of niosomes is made up of three moieties: hydrophilic, amphiphilic, and lipophilic, which can accommodate several drug molecules with a wide range of solubilities. Niosomes are preferred over liposomes because of the non-ionic surfactant's higher stability, lower cost, and ease of storage compared to phospholipids. Surfactants do not require any particular handling or storage conditions. Niosomes act as a controlled and targeted drug delivery system that reduces toxicity and increases drug penetration into target tissues. The review article focused on several ideas including advantages and disadvantages of niosomal preparations, niosome characteristics, and its application.
Keywords
Downloads
-
1FullTextPDF
Article
Introduction
Most of the unstudied drugs have low pharmacological properties, which considerably limits their therapeutic value, and 40% of commercially available pharmaceuticals are considered to contain these flaws. Therefore, there is a significant need for methods to enhance drug delivery. Nanotechnology has been widely used in pharmaceutics recently as a method to combat multi-drug resistance (MDR), improve biodistribution, and lower toxicity.1,2 New therapeutic approaches are constantly being developed to treat various diseases found all over the world. Low solubility and permeability, which results in poorer systemic absorption and bioavailability are the main issues with drugs.3,4 The non-specific & non-targeted distribution of these medicinal compounds which causes excessive toxicity and serious adverse effects is another important problem.5,6 Because they have a low therapeutic index, a short half-life, and no acceptable dose frequency that has a minimum therapeutic window or a shorter half-life or therapeutic moiety, numerous diseases do not have treatment.7,8
Novel drug delivery systems are more effective at delivering drugs to precise target areas and improving outcomes. Novel delivery system by using lipid vesicles it can deliver the drug in membrane biology, immunology, diagnostics, and genetic engineering research.9,10 Additionally, vesicles can be employed in modelling for the delivery of active pharmaceutical ingredients (API) across biological membranes and into the target region. In order to successfully tackle issues including limited permeability, low solubility, adverse effects, and systemic toxicity, many nano formulations have been developed to deliver these moieties.11 For the therapy of many illnesses, solid lipid nanoparticles (SLN), metal nanoparticles, lipid nanocarriers (niosomes and liposomes), and metal nanoparticles (gold, iron, and silver) are being widely used.12-15 In nanoparticles, drug molecules are loaded with some insert molecules or tagged passively, while in lipid nanocarriers, hydrophilic and lipophilic drugs are entrapped in hydrophilic aqueous core and hydrophobic lipid bilayer, individually.16-18 To transport hydrophobic or hydrophilic medicinal moieties, crucial nano formulation vesicles were formulated in 1961.19
Niosomes represent another novel drug delivery method, which can transport both lipid- and water-soluble drugs that are lipophilic and hydrophilic in either an aqueous layer or a bilayer membrane.20,21 Compared to liposomes, niosomes are more stable.22 Niosomes, which contain non-ionic surfactants, prolong the circulation of the carriers, delivering the drug to the targeted organs including liver, brain, and tumors.23,24 When using affordable raw materials, niosomes are more stable, reproducible, and storage-friendly than liposomes (non-ionic surfactant). Additionally, it has a clear advantage over alternative formulations for controlled or sustained release applications because of its inert, biodegradable, greater encapsulation effectiveness, and non-immunogenic qualities.25 (Figure 1)26
Niosomes
Primarily in 1975, cosmetic company Loreal developed niosomes. Niosomes are non-ionic surfactants made with self-aggregation and its bilayer of vesicles. After many years of investigation, it has been used as delivering drug device. They can easily deliver therapeutic proteins, genes and therapeutic molecules.27,28 Lipidsoluble molecules are incorporated in the lipophilic layer where drug can be loaded into the hydrophilic core as mentioned earlier.29 Hence, the formulation, classification, criteria, characterization of niosomes, and method of classification have been discussed here.30,31
Types of Niosomes
Niosomes can be divided into three kinds based on the quantity of lamellar layers or size - Large Unilamellar Vesicles (LUV, size=0.10 m), Multilamellar Vesicles (MLV), and Small Unilamellar Vesicles (SUV, size=0.025-0.05 m).11,32
Small unilamellar vesicles (SUV)
Homogenization, microfluidization and sonication, are the methods used to prepare SUVs from MLVs. SUVs are 10–100 nm in diameter. They are disposed to fusion and accumulation, have high thermodynamic instability. Their entrapped volume is minute, and the aqueous solute entrapment quantity is equally low.33,34
Large unilamellar vesicles (LUV)
LUVs are created through the utilization of both the ether injection technique and the reverse phase evaporation (REV) method.35 In order to entrap hydrophilic drug, LUV niosomes have a large aqueous section and have only one two-layer structure surrounding the aqueous core. They were used in the 1970’s, but a challenging LUV production process that frequently lasted for several days severely slowed down the advancement in some areas of model membrane research. LUV formulation has been widely used in the 1980’s.36 This type of niosome can encapsulate large amounts of bioactive chemicals at a very low cost.37
Multilamellar vesicles (MLV)
MLVs are larger than 50 nm, containing more than one layer (or lamella). Multilamellar vesicles (MLVs) are formulated by an exterior vesicle with a diameter higher than 1000 nm. MLVs are formulated by a transmembrane pH gradient and thin film hydration method (TFH). In this process, nonionic surfactant and the lipophilic drug are dissolved in an organic solvent in a rotary evaporator. The solution evaporates under pressure, and the solvent is evaporated by slightly heating it. This is hydrated using tris (hydroxymethyl) aminomethane (Tris) buffer or Phosphate buffer to produce niosomes. Most of the time, it involves the use of energy. The film that forms inside the flask is typically removed gradually during the agitation in a vortex using glass beads to maintain the MLV layers.38
Proniosome: These dry, powdered compositions are water-soluble and surfactant-coated. It is rehydrated while being used, which helps to get over niosome limitations such as low therapeutic moieties, vesicles fusing together, and aggregation.39.
Aspasome: Ascorbyl palmitate (ASP), more negatively charged lipids such as diacetyl phosphate, and cholesterol are used in the preparation. It improves transdermal drug administration and lessens the negative effects brought on by reactive oxygen species (ROS). These are vesicles with several layers.10
Bola niosomes: By adopting a niosomal formulation, Bola C16, also known as Bola C16 was developed which is a mixture of cholesterol, span 80, and 1-aza-18-crown-6 surfactants. This is employed to increase permeability in transdermal medication administration applications.40
Discome: Poly-24-oxyethylene ether (Solulan C24) cholesteryl and a small amount of cholesterol are used to organise MLVs. Their size spans from 11 to 60 m, and it develops a sustained release formulation for ocular distribution.41
Polyhedral niosomes: By substituting polyoxyethylene cholesteryl ether or hexadecyl diglycerol ether for non-ionic surfactants, these formulations are made without cholesterol (C16G2). Due to their lack of specific structure, vesicles can capture particles that are soluble in water.42
Vesicles in w/o emulsion system: It has been done to create niosomal vesicles as a water-in-oil emulsion. Cholesterol, span 60, and solulan C24 are only a few of the substances used in the creation of vesicles (poly-24-oxyethylene cholesteryl ether). These formulations provide peptides and enzymes, but are sensitive to environment.43
Niosomes in carbopol gel: Niosomes are created utilizing non-ionic surfactant and appropriate cholesterol. The Carbopol gel is then added to the created niosomes. The mucoadhesion characteristic is improved by this preparation.44
Components of Niosomes
To prepare niosomes, the following components are needed. Niosomes are made up of cholesterol or its derivatives, nonionic drug surfactants, and ion amphiphiles. Both hydrophilic and lipophilic drugs can be entrapped by niosomes. Hydrophobic drugs are contained in a comparable core as a two-layer lipophilic zone, while hydrophilic drugs are enclosed in the latter. The right amount of cholesterol is added to establish a stable formulation by interaction with nonionic surfactants.45
Nonionic surfactants
The most significant molecules are surface-active agents, sometimes referred to as surfactants. Surfactants are amphiphilic because their structures have hydrophilic heads and hydrophobic tails.46,47 They are divided into anionic, cationic, amphoteric, and nonionic groups based on the hydrophilic groups they contain. They have a wide range of polar and non-polar interactions due to their amphiphilic nature. Chemical, organic, and physical structures cannot exist without surfactants. Because they work well to formulate artificial lipid bilayer membranes, phospholipids and surfactants are frequently used in the delivery of formulation.48 Amphiphilic compounds exposed to an aqueous environment at a concentration greater than their critical micelle concentration (CMC) result in the formation of various nanostructures (CMC). Nonionic surfactants make up a substantial portion of niosomes because of their enhanced biocompatibility in comparison to other surfactants.
Hydrophilic-Lipophilic balance (HLB): Cosmetic and pharmaceutical companies frequently use the Hydrophilic-Lipophilic balance (HLB) scale which ranges from 1 to 14, the number of amphiphilic combinations, and the classification it produces. Water in oil (W/O) and oil in water (O/W) emulsions are two examples of formula-based surfactant selection. Griffin's number of HLB is the most common concept among surfactant users. Ester surfactants and non-toxic surfactants are most commonly utilized in this area. The HLB measure spans from 0 to 20, and the HLB number indicates the number of surfactants used, such as detergents (HLB: 13 to 15), wetting factors (HLB: 4 to 6), o/w emulsifiers (HLB: 7 to 9), and detergents (HLB: 8 to 18) when there are no emulsifiers present. Niosome synthesis is not appropriate for surfactants with an HLB number of 14 to 17.49
Critical packing parameter (CPP): The morphologies of the forming aggregates can be predicted using the surfactant molecule's nominal geometric properties. The CPP parameter is helpful in preventing the construction of self-assembled amphiphiles. The type of aggregation induced by surfactants is defined by this parameter (for example, as spherical or cylindrical micelles, vesicles, or flexible or fixed bilayers).50 The area of the polar head group (a0), the volume of the non-polar group (V), and the critical non-polar group length (lc) are used in the equation to compute the CPP value.51,52 The membrane composed of nonionic surfactants with high and low levels of HLB values ( Figure 2)53
Cholesterol
Cholesterol is a primary component of niosomes that has the potential to influence their physicochemical properties and overall stability.54 Cholesterol is the main component of niosomes, cholesterol and surfactant, are added in specific proportions to give dispersions and gels.55 From formulation perspective, vesicles' particle diameter is an important feature. It has been demonstrated that cholesterol, regardless of the type of surfactant utilized, significantly affects the particle dimension of niosomes. However, the kind of nonionic surfactant was substantially correlated with the effect of cholesterol percentage on the size of niosomes.56
Preparation of Niosomes
Similar to liposomes, niosomes can be widely classed based on their lamellarity. The bilayered vesicles might be Small Unilamellar Vesicles (SUV), Large Unilamellar Vesicles (LUV), or Multi-Lamellar Vesicles (MLV). For the optimization of certain delivery needs, different niosome sizes may be required, and a variety of techniques are employed to develop these nanocarrier systems. Depending on the desired vesicle size, size distribution, number of bilayers, aqueous phase entrapment effectiveness, and needed permeability of the vesicle membranes, niosomes can be made in a variety of ways. In general, the production of niosomes involves hydration of a surfactant and lipid mixture in an aqueous medium at a high temperature (often >60o C), followed by an optional size reduction procedure and finally the formulation of a colloidal dispersion.42
Preparation of Multilamellar Vesicles (MLV)
Thin-film hydration technique
This method is frequently used to formulate niosomes. As the initial step in this method, cholesterol and nonionic surfactants are added to an appropriate organic solvent (chloroform, ethanol, etc.). The rotatory evaporator is used to formulate a thin film. The formed film is hydrated using buffer or water as a hydrating medium, keeping temperature above the gel-liquid transition point to produce a milky suspension of niosomes.57,58 (Figure 3)53
Transmembrane pH gradient drug uptake
This approach is suitable for hydrophobic and ionizable drugs. Initially, a mixture of lipids, surfactant, and cholesterol is dissolved in chloroform. To create a thin film on the interior surface of a round-bottom flask, the solvent is subsequently evaporated under reduced pressure. The film is then hydrated using a vortex mixer with a 300 mM citric acid solution at pH 4.0. After subjecting the mixture to three cycles of freezing and melting, followed by three rounds of sonication, multilamellar vesicles are formed. The drug-containing aqueous solution is introduced into the vortexed niosome suspension. Upon adding a 1 M disodium phosphate solution, the sample's pH is adjusted to fall within the range of 7.0 to 7.2. The mixture is then heated at 60°C for 10 minutes to generate multilamellar vesicles. These vesicles possess a neutral exterior and can encapsulate both protonated and unprotonated forms of the payload. Due to the porous nature of the niosome membrane, the unprotonated form of the payload can permeate it. As the contents of the vesicle are acidic, the drug becomes protonated inside and remains in this form. The balance of drug concentration inside and outside the vesicle membrane must be achieved before diffusion ceases.59
Preparation of Small Unilamellar Vesicle (SUV)
Microfluidization method
Using microchannels with a diameter between 5 m and 500 m to regulate material flow are referred to as microfluidic methods. Within an interaction chamber, a flow of lipids suspended in either ethanol or isopropanol is directed through purpose-built microchannels, where it comes into contact with an aqueous stream at exceptionally high velocities. Niosomes are created through a process involving the self-assembly of surfactants, which occurs as they locally diffuse in contact with the aqueous phase, and this process is continually facilitated by the ongoing axial mixing of the two phases. When niosomes are produced using this technology, they are highly reproducible and homogenous, and they produce vesicles with a diameter of just 0.200 nm.60,61 (Figure 4)53
Sonication
In order to sonicate a mixture of surfactant and cholesterol in a scintillation vial, an aqueous phase containing a drug is added. A sonic probe is used to homogenize the mixture for up to three minutes at 60°C. Small and uniformly sized vesicles are developed. The phase transition temperature is exceeded, and sonication is performed for more than 20 minutes.62,63 (Figure 5)64
Preparation of Large Lamellar Vesicle
Reversed-Phase Evaporation (REV)
The reverse-phase evaporation (REV) method is used to formulate LUVs, cholesterol, and surfactants, dissolved in an organic solvent. The aqueous medium (which contains the drug) is combined with the organic medium, and the mixture is then sonicated to formulate an emulsion. Next, the organic phase is slowly removed using a rotary vacuum evaporator at a temperature of around 40 to 60°C. Until the hydration is finished, the evaporation will continue. During the evaporation of an organic dissolvent, LUVs are formulated.65,66 (Figure 6)67
Ether Injection
This approach involves gently injecting the surfactants and additives, which are dissolved in diethyl ether and heated to steady temperature above the boiling point of the organic solvent, through a needle into an aqueous drug solution. Using a rotary evaporator, the organic solvent is evaporated. Single-layered vesicles are formed as a result of vaporisation.68,69(Figure 7)53
Miscellaneous Techniques
Emulsion Formation
The first step in this process involves forming an oil-in-water (o/w) emulsion from an aqueous solution containing the drug, cholesterol, and surfactant.70 Niosomes grow and become disseminated in the aqueous phase as a result of organic solvent evaporating.
Lipid Injection
The lipid injection method is environmentally friendly because it does not use any surplus organic phases. Melting a combination of surfactant and lipid is followed by injection into a hot, rapidly agitated deug aqueous solution.11,71
Niosome Characteristics
Particle Size (PS) and Polydispersity Index (PDI)
The diameter of niosomes is measured using laser light scattering and transmission electron microscopy (TEM). Niosomes may have a spherical shape. The size of niosomes has also been measured using ultracentrifugation, molecular sieve, photon correlation microscopy, chromatography, and optical microscopy.72,73 PS is necessary for niosomes to cross biological barriers like the blood-brain barrier (BBB). Small particles have a capacity to transfer drugs to the brain or for phagocytosis by macrophages in cases of diseases like HIV/AIDS, TB, or malaria. This makes crossing the BBB crucial for targeted medication administration.74,75 The formulation includes the measurement of niosome particle size distribution using the particle distribution index (PDI), with a small PDI often chosen to allow for precise assessment of particle behavior.76 The most popular method for determining particle size and polydispersity is photon correlation spectroscopy (PCS), which measures a dispersion's PDI.77 Dynamic light scattering (DLS) or quasi-elastic light scattering (QELS) are other methods that are frequently employed. With the help of solvent molecules, which scatter the applied light, Brownian motion of the particles is screened using DLS. The device calculates the scattering light's rate, intensity, and time-dependent variation. They are connected to how quickly dispersed particle diffusivity occurs. The difference between the particles determine the amount of light scattering and the solvent's refractive index. Smaller particles diffuse more quickly than larger particles. This method is quick, and reliable. It provides the average particle size of a dispersion and estimates the amount of light that has been dispersed. When doing particle size analysis on natural niosomal preparations, DLS is advantageous since it may be utilized to evaluate a broad size range ranging from a few nanometers to several micrometers.78 This approach, though, has drawbacks. The impact of particle solvation cannot be eliminated from the results because DLS cannot distinguish between individual particles and particle aggregates.
The size determined by DLS is referred to as a hydrodynamic diameter, and DLS particle size analysis is very sensitive to particulate contamination.79
Morphology
It is possible to determine shape, size, and structure using essential morphological factors. A brief description of the resulting formulation's surface morphology is given. Utilizing tools like transmission electron microscopy (TEM), atomic force microscopy (AFM), and field emission scanning electron microscopy (FESEM) allows for the accurate size and shape imaging of complicated structures (TEM). Confocal laser scanning microscopy (CLSM) helps distinguish the hydrophilic and lipophilic layer in the niosome as well as the medicinal moiety that is captured by the application of a suitable dye. Leica microscopy is used to investigate the formation of a ring-like structure in niosomes of basic drug design.80,81
Zeta Potential (ZP)
According to the formulation's ingredients, the ZP of niosomal dispersions resemble the total of surface charges. It is essential to analyze the surface charge of the niosomes in order to predict the long-term stability of the technology, the in vivo performance, and the biological chance of the carriers. In comparison to neutral and mildly charged niosomes, those that have a significant negative or positive charge show little agglomeration in a dispersion. This is related to neutral dispersions' absence of electrostatic repulsive forces. Dynamic light scattering (DLS) is employed to quantify fluctuations in the intensity of scattered light arising from the mobility of niosomes due to the influence of an electric field applied during the measurement of zeta potential (ZP). The surface charge of niosomes governs their mobility, causing fluctuations in the scattered light intensity.82,83
Entrapment Efficiency
The percentage of drug included in the formulation is often calculated using this method. Drug delivery depends mainly on entrapment efficiency, which can also be used to calculate the dosage frequency of formulations. Furthermore, increasing the drug loading in the formulation can effectively lower the therapeutic moiety's dose frequency. Among the several described methods, the dialysis bag approach is the one that is most frequently utilized. The produced formulation is kept in the dialysis tube and sealed on both sides before being added to the dialysis medium and left there for 24 hours at a thermostatic temperature of 350.5°C. When the unentrapped drug exits the dialysis membrane, its concentration is often evaluated using high-performance liquid chromatography (HPLC) at a preset wavelength or UV visible spectroscopy to determine how much of the drug is still trapped. The ratio of the amount dialyzed to the total amount integrated is used to determine the entrapment efficiency of drug-loaded niosomes.84 Entrapment efficiency can also be measured using fluorescence spectroscopy.85
Wherein, CTotal is the amount of drug incorporated, Cout is the amount of drug unentrapped
In Vitro Drug Release
Drug concentration, hydration volume, stiffness, and bilayer state are among the fundamental parameters that might affect in vitro drug release performance of niosomes. Dialysis membrane techniques are typically used to study the release of drug from niosomes. Purified niosomal suspension is placed in a dialysis bag, closed at both ends, and placed in a container, such as a beaker containing phosphate buffered saline, and kept at 37°C with agitation. At predefined intervals, samples are withdrawn and replaced with the same volume of new medium. To determine the concentration of drug delivered over time, these samples are subsequently examined using the quantitation procedures.86,87,88 Other alternative techniques involving the use of Franz diffusion cells have been documented, and they generally follow comparable protocols and procedures.
Stability
A formulated formulation's efficacy over a specific time period is provided by stability data. Storage-related problems such as aggregation, fusion, photodegradation, and drug leakage from these vesicles are the significant drawbacks of these vesicles. Only a small number of niosomal formulations are reported to exhibit more than 60% entrapment efficiency and about 90% retention following 30 days of stability testing. The lack of a significant change in a formulation's structure, charge, size, and other characteristics is another sign of stability.89,90
An essential factor in determining whether the drug is adsorbed on the niosome surface which could be a predictor of burst release and encapsulation efficiency is the surface makeup of niosomes.89 This is accomplished by using the SEM and EDX methods, or elemental dispersive spectroscopy. The method has been successfully applied in liposomes to detect whether successful drug loading occurred, while not being extensively employed in niosomes.91,92,93 X-ray photoelectron spectroscopy (XPS) presents a potentially valuable method for analyzing the surface elemental composition of niosomes. While there is no known application of XPS specifically in niosomes, its successful application in liposomes has been documented, suggesting the possibility of extrapolating this technique to study niosomes.94
Advantages of Niosomes
- The niosome serves as a reservoir for the controlled and slow release of drugs.
- Niosomes are stable and osmotically active. Additionally, compared to liposomes, the medication has substantially higher stability over extended periods of storage.
- The medication compliance is being enhanced in one of the following ways: delaying drug clearance, shielding the drug from the biological environment, or limiting the drug's effects to the intended site.
- Niosomes have low toxicity and are more stable because commonly used nonionic surfactants are biocompatible, biodegradable, and non-immunogenic. Niosomes are highly compatible with biological systems and have low toxicity.84,95,96
Limitation of Niosomal Drug Delivery System
Toxicity of niosomes has not received adequate attention. Various surfactants (other than niosomes) have been studied for their potential toxicity. According to the research, nonionic surfactants have higher compatibility and lower toxicity when compared to other types of surfactants, and ester surfactants are less hazardous than ether surfactants (because of the impact of enzymes on the ester link). The research findings demonstrate that polyoxyethylene chain length increases cytotoxicity, while increase in alkyl chain length of surfactants results in a decrease in toxicity. The sterilization of niosomes, instability of aqueous suspension of niosomes (which may unintentionally result in a decrease in the shelf life), and, in some situations, the cost are some problems that restrict the use of niosomes in drug delivery systems.97 98,99
Comparison of Niosomes Versus Liposomes
Niosomes and liposomes are bilayer vesicles, but composition and chemical characteristics differ. Liposomes are made up of phospholipids, while the niosomes are nonionic surfactants. Both structures can be utilized in continuous and targeted medication delivery systems and perform equally well as carriers. According to studies, both systems prolong the entrapped drug's circulation period and changes distribution and metabolic stability. Because of all these similarities, niosomes are considered as an alternative to liposomes since they have advantages over them. The phospholipids that make up liposomes are chemically unstable, while the niosomes exhibit greater chemical stability. Niosomes are less expensive than liposomes, and liposomes need particular handling, storage, and purification techniques for phospholipids.100,101,10
Conclusion
Non-ionic surfactants, which are safe and have improved stability, are a crucial component in the creation of niosomes, as was covered in the review paper. Niosomes are one of the best carrier systems for delivering medications with fewest negative effects, as has been established. Minimal manufacturing costs, purity, chemical stability, and low toxicity are a few advantages of niosomes making them the best option for selection. In the end, it can be said that niosomes are the best delivery systems for both hydrophobic and hydrophilic medications since they can do so with the least amount of adverse effects, improved targeting, and increased bioavailability of the drug at the site of action.
Conflict of Interest
None
Supporting File
References
- Sun Y, Ma X, Hu H. Application of nano-drug delivery system based on cascade technology in cancer treatment. Int J Mol Sci 2021;22(11):5698.
- Yousefi M, Dadashpour M, Hejazi M, Hasanzadeh M, Behnam B, de la Guardia M, et al. Anti-bacterial activity of graphene oxide as a new weapon nanomaterial to combat multidrug-resistance bacteria Mater Sci Eng C Mater Biol Appl 2017;74: 568–581.
- Hauss D. Oral Lipid-Based formulations: Enhancing the Bioavailability of Poorly Water Soluble Drugs. Informa Healthcare 2007;170: 1-304.
- Lindenberg M, Kopp S, Dressman J. Classification of orally administered drugs on the World Health Organization Model list of Essential Medicines according to the biopharmaceutics classification system. Eur J Pharm Biopharm 2004;58(2): 265-78.
- Riley RJ. An evaluation of the importance of metabolic activation and detoxication in drug toxicity. Library British 2010;10:441-540.
- Wen H, Jung H, Li X. Drug delivery approaches in addressing clinical pharmacology-related issues: opportunities and challenges. AAPS J 2015;17 (6):1327–1340.
- Guengerich FP. Mechanisms of drug toxicity and relevance to pharmaceutical development. Drug Metab Pharmacokinet 2011;26(1):3-14.
- Lewis R. Current concepts in antifungal pharmacology. Mayo Clin Proc 2011;86(8):805-817.
- Barani M, Mirzaei M, Torkzadeh-Mahani M, Lohrasbi-Nejad A, Nematollahi M. A new formulation of hydrophobin-coated niosome as a drug carrier to cancer cells. Mater Sci Eng C Mater Biol Appl 2020;113:110975.
- Paolino D, Cosco D, Muzzalupo R, Trapasso E, Picci N, Fresta M. Innovative bola-surfactant niosomes as topical delivery systems of 5-fluorouracil for the treatment of skin cancer. Int J Pharm 2008;353(1):233–242.
- Moghassemi S, Hadjizadeh A. Nano-niosomes as nanoscale drug delivery systems: an illustrated review. J Control Release 2014;185:22-36.
- Jahangirian H, Kalantari K, Izadiyan Z, Rafiee Moghaddam R, Shameli K, Webster T. A review of small molecules and drug delivery applications using gold and iron nanoparticles. Int J Nanomedicine 2018;14:1633–1657.
- Torchilin VP. Targeted pharmaceutical nanocarriers for cancer therapy and imaging. AAPS J 2007;9: 128-147.
- Wang M, Thanou M. Targeting nanoparticles to cancer. Pharmacol Res 2010;62(2):90-99.
- Wong H, Bendayan R, Rauth M, Li Y, Wu X. Chemotherapy with anticancer drugs encapsulated in solid lipid nanoparticles. Adv Drug Deliv Rev 2007;59(6):491-504.
- Akbarzadeh I, Shayan M, Bourbour M, Moghtaderi M, Noorbazargan H. Preparation, Optimization and In-Vitro Evaluation of Curcumin-Loaded Niosome@ calcium alginate nanocarrier as a new approach for breast cancer treatment. Biology 2021;10(3):4064-4071.
- Ashley J, Quinlan J, Schroeder A, Suckow A, Pizzuti V, Kiziltepe T. Dual carfilzomib and doxorubicin–loaded liposomal nanoparticles for synergistic efficacy in multiple myeloma. Mol Cancer Ther 2016;15(7):1452-59.
- Khan DH, Bashir S, Khan MI, Figueiredo P, Santos H, et al. Formulation optimization and in vitro characterization of rifampicin and ceftriaxone dual drug loaded niosomes with high energy probe sonication technique. J Drug Deliv Sci Technol 2020;58:1-8.
- Marianecci C, Di Marzio L, Rinaldi F, Celia C, Paolino D, Alhaique F, et al. Niosomes from 80s to present: the state of the art. Adv Colloid Interface Sci 2014;205:187-206.
- Bangham A, Surrogate D. Cells or Trojan Horses: The Discovery of Liposomes. Bioessays 1995; 17:1081–1088.
- Singh D, Pradhan M, Nag M, Singh MR. Vesicular system: Versatile carrier for transdermal delivery of bioactives. Artif Cells Nanomed Biotechnol 2015;43(4):282–290.
- Kumar G, Rajeshwarrao P. Nonionic surfactant vesicular systems for effective drug delivery An overview. Acta Pharmaceutica Sinica B 2011;1 (4):208–219.
- Shakya V, Bansal B. Niosomes K: A novel trend in drug delivery. Int J Res Deliv Pharm Life Sci 2014;3:1036–1041.
- Kazi KM, Mandal AS, Biswas N, Guha A, Chatterjee S, Behera M, et al. Niosome: A future of targeted drug delivery systems. J Adv Pharm Technol Res 2010;1(4):374-380.
- Azmin MN, Florence AT, Handjani RM, Stuart JF, Vanlerberghe G, Whittaker JS. The effect of non-ionic surfactant vesicle (niosome) entrapment on the absorption and distribution of methotrexate in mice. J Pharm Pharmacol 1985;37:237-242.
- Pandey S, Shah K, Maulvi F, Desai D, Gupta A, et al. Topical delivery of cyclosporine loaded tailored niosomal nanocarriers for improved skin penetration and deposition in psoriasis: Optimization, ex vivo and animal studies. J Drug Deliv Sci Technol 2021;63:102441.
- Shilpa S, Srinivasan B, Chauhan M. Niosomes as vesicular carriers for delivery of proteins and biologicals. Int J Drug Deliv 2011;3:14-24.
- Puras G, Mashal M, Zárate J, Agirre M, Ojeda E, Grijalvo S, et al. A novel cationic niosome formulation for gene delivery to the retina. J Control Release 2014;174:27-36.
- Maurer V, Altin S, Ag Seleci D, Zarinwall A, Temel B, Vogt PM, et al. In-vitro application of magnetic hybrid niosomes: targeted siRNA-delivery for enhanced breast cancer therapy. Pharmaceutics 2021;13(3):394-404.
- Samed N, Sharma V, Sundaramurthy A. Hydrogen bonded niosomes for encapsulation and release of hydrophilic and hydrophobic anti-diabetic drugs: An efficient system for oral anti-diabetic formulation. Appl Surf Sci 2015;449:567-73.
- Ag Seleci D, Seleci M, Walter M, Stahl G, Scheper T. Niosomes as nanoparticular drug carriers: Fundamentals and recent applications. J Nanomater 2016;2016:1-10.
- Johnson S, Buttress N. The osmotic insensitivity of sonicated liposomes and the density of phospholipid-cholesterol mixtures. Biochim Biophys Acta 1973;307:20–26.
- Mui B, Cullis P, Evans E, Madden T. Osmotic properties of large unilamellar vesicles prepared by extrusion. Biophys J 1993;64:443–453.
- Banke B, Thakre N. Design of Gatifloxacin Niosomes by film hydration method. Int J Soc Impact 2020;113(5):110975-982.
- Mui B, Hope M. Formation of large unilamellar vesicles by extrusion: Liposome technology Novel vesicular drug delivery systems: a review. Pharmaceutics 2018;44(4):77–88.
- Ray SK, Bano N, Shukla T, Upmanyu N, Pandey SP, Parkhe G. Noisomes: as novel vesicular drug delivery system. Journal of Drug Delivery Therapeutics 2018;8(7):335–341.
- Myneni G, Radha G, Soujanya G. Novel vesicular drug delivery systems: a review. J Pharm Res 2018; 4(70):123-41.
- Coutinho CC, Dos Santos E, Mansur C. Niosomes as nano-delivery systems in the pharmaceutical field. Crit Rev Ther Drug Carrier Syst 2016;33(2): 195-212.
- Vora B, Khopade A, Jain N. Proniosome based transdermal delivery of levonorgestrel for effective contraception. J Control Release 1998;54(2): 149-65.
- Gopinath D, Ravi D, Rao B, Apte S, Renuka D, Rambhau D. Ascorbyl palmitate vesicles (Aspasomes): formation, characterization and applications. Int J Pharm 2004;271(1):95-113.
- Uchegbu F, Vyas S. Non-ionic surfactan based vesicles (niosomes) in drug delivery. Int J Pharm 1998;172(1):33-70.
- Arunothayanun P, Uchegbu F, Craig D, Turton J, Florence A. In vitro/in vivo characterization of polyhedral niosomes. Int J Pharm 1999;183(1): 57-68.
- Gharbavi, Amani M, Kheiri-Manjili J, Danafar H, Sharafi A. Niosome: a promising nanocarrier for natural drug delivery through blood-brain barrier. Adv Pharmacol Pharm Sci 2018;2018:1-10.
- Ning M, Guo Y, Pan H, Chen X, Gu Z. Preparation, in vitro and in vivo evaluation of liposomal/ niosomal gel delivery systems for clotrimazole. Drug Dev Ind Pharm 2005;31(4):1-10.
- Xuemei G, Minyan w, Suna H, Wei N. Advances of non-ionic surfactant vesicles (niosomes) and their application in drug delivery. Pharmaceutics 2019;11(2):1-20.
- Imdad A, Salim S, Farid A, Shafi U, Imkan I, Kashif N. Synthesis of long-tail nonionic surfactants and their investigation for vesicle formation, drug entrapment, and biocompatibility. J Liposome Res 2020;30(3):255-62.
- Chen S, Hanning S, Falconer J, Locke M, Wen J. Recent advances in nonionic surfactant vesicles (niosomes): Fabrication, characterization, pharmaceutical and cosmetic applications. Eur J Pharm Biopharm 2019;144:18-39.
- Bhardwaj S, Bhatia S. Development and characterization of niosomal gel system using Lallementia royaleana Benth. mucilage for the treatment of rheumatoid arthritis. Iran J Pharm Res 2020;I9:465-78.
- Griffin W. Classification of surface-active agents by “HLB”. J Soc Cosmetic Chem 1947;1:311–326.
- Khoee S, Yaghoobian M. Niosomes: A novel approach in modern drug delivery systems. Nanostructures for drug delivery. Elsevier Inc; 2017. p. 207–237.
- Kobierski J, Wnętrzak A, Chachaj-Brekiesz A, Dynarowicz-Latka, P. Predicting the packing parameter for lipids in monolayers with the use of molecular dynamics. Colloids Surf B Biointerfaces 2021;11(2)298-305.
- Israelachvili J, Mitchell D, Ninham B. Theory of self-assembly of hydrocarbon amphiphiles into micelles and bilayers. J Chem Soc Faraday Trans 1976;2(72):1525–1568.
- Mujeeb S, Sailaja A. Formulation of ibuprofen loaded niosomal gel by different techniques for treating rheumatoid arthritis. J Bionanosci 2017;11:169–176.
- Estupinan OR, García PM, Blanco-Lopez MC, Matos M, Gutierrez G. Vitamin D3 loaded niosomes and transfersomes produced by ethanol injection method: identification of the critical preparation step for size control. Food 2020;9(10):1-9.
- Bartelds R, Nematollahi M, Pols T, Stuart MCA, Pardakhty A, Asadikaram G, et al. Niosomes, an alternative for liposomal delivery. PLoS One 2018;13(4):e0194179.
- Ruwizhi N, Aderibigbe A. The efficacy of cholesterol-based carriers in drug delivery. Molecules 2020;25(18):4330.
- Nowroozi F, Almasi A, Javidi, J, Haeri A, Dadashzadeh S. Effect of surfactant type, cholesterol content and various downsizing methods on the particle size of niosomes. Iran J Pharm Res 2018;1(7):1-15
- Bagheri A, Chu B, Yaakob H. Niosomal drug delivery systems: Formulation, preparation and applications. World Appl Sci J 2014;32:1671– 1685.
- Muzzalupo R, Tavano L, La Mesa C. Niosomal plumbagin with reduced toxicity and improved anticancer activity in BALB/C mice. J Pharm Pharmacol 1996;48(11):1128-32.
- Mayer LD, Bally MB, Hope MJ, Cullis PR. Uptake of antineoplastic agents into large unilamellar vesicles in response to a membrane potential. Biochem Biophys Acta Biomembr 1985;816(2):294-302.
- Mujoriy R, Bodla S, Dhamande K, Singh D, Patle L. Niosomal drug delivery system: The magic bullet. J Appl Pharm Sci 20111;7:20–23.
- Szoka F, Papahadjopoulos D. Procedure for preparation of liposomes with large internal aqueous space and high capture by reverse-phase evaporation. Proc Natl Acad Sci USA 1978;75 (9):4194–4198.
- Bendas E, Abdullah H, El-Komy, M, Kassem M. Hydroxychloroquine niosomes: a new trend in topical management of oral lichen planus. Int J Pharm 2013;458:287–295.
- Talegaonkar S, Mishra P, Khar R, Biju S. Vesicular systems: An overview. Indian J Pharm Sci 2006; 68:141-155
- . Balasubramaniam A, Kumar V, Pillai K. Formulation and in vivo evaluation of niosomeencapsulated daunorubicin hydrochloride. Drug Dev Ind Pharm 2002;28(10):1181–1193.
- Monavari S. The inhibitory effect of Acyclovir loaded nano-niosomes against herpes simplex virus type-1 in cell culture. MJIRI 2013;33(3):17- 19.
- Ali M, Abdel Motaal A, Ahmed M, Alsayari, A, El-Gazayerly O. An in vivo study of Hypericum perforatum in a niosomal topical drug delivery system. Drug Deliv 2018;25:417–425.
- Weng, H, Liu X, Ren Y, Li Y, Li X. Fingolimod loaded niosomes attenuates sevoflurane induced cognitive impairments. Biomed Microdevices 2022;24:1–11.
- Ijeoma F, Uchegbu, Suresh P, Vyas. Noionic surfactant based vesicles (niosomes) in drug delivery. Int J Pharm 1998;172:33–70.
- Biju S, Talegaonkar S, Misra P, Khar K. Vesicular systems: A review. Indian J Pharm Sci 2006;68:1418153.
- Pardakhty A, Varshosaz, J, Rouholamini A. In vitro study of polyoxyethylene alkyl ether niosomes for delivery of insulin. Int J Pharm 2007;328(2): 130–141.
- Talegaonkar S, Mishra P, Khar R, Biju S. Vesicular systems: An overview. Indian J Pharm Sci 2006;68(5):141-48.
- Le Maire M, Champeil P, Moller JV. Interaction of membrane proteins and lipids with solubilizing detergents. Biochem Biophys Acta Biomembr 2000;1508(1):86–111.
- Kuo Y, Chung G. Physicochemical properties of nevirapine-loaded solid lipid nanoparticles and nanostructured lipid carriers. Colloids Surf B 2011;83(2): 299–306.
- Lu C, Zhao Z, Wong L, Cai J, Peng L, Tian L, et al. Current approaches to enhance CNS delivery of drugs across the brain barriers. Int J Nanomedicine 2014;9(1):2241–2257.
- Priyanka K, Singh S. A review on skin targeted delivery of bioactives as ultradeformable vesicles: Overcoming the penetration problem. Curr Drug Targets 2014;15(2):184–198.
- Sezgin Z, Yuksel N. Investigation of formulation variables and excipient interaction on the production of niosomes. AAPS PharmSciTech 2012;13: 826–835.
- Sergeev B, Klabunde J. Experimental Techniques. In: Nano chemistry. 2nd ed. Oxford, UK: Elsevier; 2013. p. 31–43.
- Winter T. Measurement of suspended particles by quasi-elastic light scattering. J Polym Sci 1983;21(12):1020-28.
- Ruckmani K, Sankar V. Formulation and optimization of zidovudine niosomes. AAPS Pharm Sci Tech 2010;11:1119–1127.
- Tangri P, Khurana S. Niosomes: formulation and evaluation. International Journal of Biopharmaceutics 2011;4(5):4995-98.
- Verma S, Singh S, Syan N, Mathur P, Valechs V. Nanoparticle vesicular systems: a versatile tool for drug delivery. J Chem Pharm Res 2010;2(2): 496-506.
- Bragagn M, Mennini N, Ghelardini C, Mura P. Development and characterization of niosomal formulations of doxorubicin aimed at brain targeting. J Pharm Pharm Sci 2012;15(1):184–196.
- Begum M, Dasari S, Sudhakar M, Lakshmi B, Manga, K Begum, et al. Development and evaluation of co-encapsulated stavudine and lamivudine niosomes for the controlled delivery. Der Pharm Sin 2014;5:1–10.
- . Firthouse S, Halith M, Wahab S, Sirajudeen M, Mohideen K. Formulation and evaluation of miconazole niosomes. Int J Pharmtech Res 2011; 3(2):1019-22.
- Mehta S, Jindal N, Kaur G. Quantitative investigation, stability and in vitro release studies of antiTB drugs in Triton niosomes. Colloids Surf B Biointerfaces 2011;87(1):173-179.
- Witika A, Walker B. Development, manufacture and characterization of niosomes for the delivery for nevirapine. Pharmazie 2019;74:91–96.
- Sreya M, Krishna A, Preparation and evaluation of diclofenac sodium niosomal formulations. J Bionanosci 2017;11:489–496.
- Wang, M, Yuan Y, Gao Y, Ma, H, Xu M, et al. Preparation and characterization of 5-fluorouracil pH-sensitive niosome and its tumor-targeted evaluation: In vitro and in vivo. Drug Dev Ind Pharm 2012;38(12):1134–1141.
- Ammar H, Ghorab M, El-Nahhas S, Higazy I. Proniosomes as a carrier system for transdermal delivery of tenoxicam. Int J Pharm 2011;405(1): 142-152.
- Abd-Elbary A, El-Laithy H, Tadros I. Sucrose stearate-based proniosome-derived niosomes for the nebulisable delivery of cromolyn sodium. Int J Pharm 2008;352(2):189-198.
- Ammar HO, Ghorab M, El-Nahhas S, Higazy IM. Proniosomes as a carrier system for transdermal delivery of tenoxicam. Int J Pharm 2011;405: 142-152.
- Nkanga C, Krause R. Encapsulation of isoniazidconjugated phthalocyanine in-cyclodextrin-in-lipo-somes using heating method. Sci Rep 2019; 9:11485-90.
- Okafor N, Nkanga I, Walker R, Noundou X, Krause R. Encapsulation and physicochemical evaluation of efavirenz in liposomes. J Pharm Investig 2020;50:201–208.
- Chen H, Torchilin V, Langer R. Lectin-bearing polymerized liposomes as potential oral vaccine carriers. Pharm Res 1996;13:1378–138.
- Shakya V. Niosomes: A novel trend in drug delivery. IJRDP 2014;3:1036-1041.
- Makeshwar K, Wasankar S. Niosomes: a novel drug delivery system. Asian J Pharm Res 2013;3: 16-20.
- Jindal K. Niosomes as a potential carrier system: a review. IJPCBS 2015;5:947-959.
- Kaur H, Dhiman S, Arora S. Niosomes: A novel drug delivery system. Int J Pharm Sci Res 2012;15: 113-120.
- Maurer V, Altin S, Seleci D, Zarinwall A, Temel B. et al. In-vitro application of magnetic hybrid niosomes: targeted siRNA-delivery for enhanced breast cancer therapy. Pharmaceutics 2021; 13(3):394-98.
- Moghaddam F, Akbarzadeh I, Marzbankia E, Farid M, Reihani, A, et al. Delivery of anticancer effect. Cancer Nanotechnol 2021;12(2): 1–35.
- Jafari-Gharabaghlou D, Pilehvar-Soltanahmadi Y, Dadashpour M, Mota A. Combination of metformin and phenformin synergistically inhibits proliferation and hTERT expression in human breast cancer cells. Iran J Basic Med Sci 2018; 21(2):1167-72.
- Sabry S, Okda T, Hasan A. Formulation, characterization, and evaluation of the anti-tumor activity of nanosized galangin loaded niosomes on chemically induced hepatocellular carcinoma in rats. J Drug Deliv Sci Technol 2021;61: 102163-78.