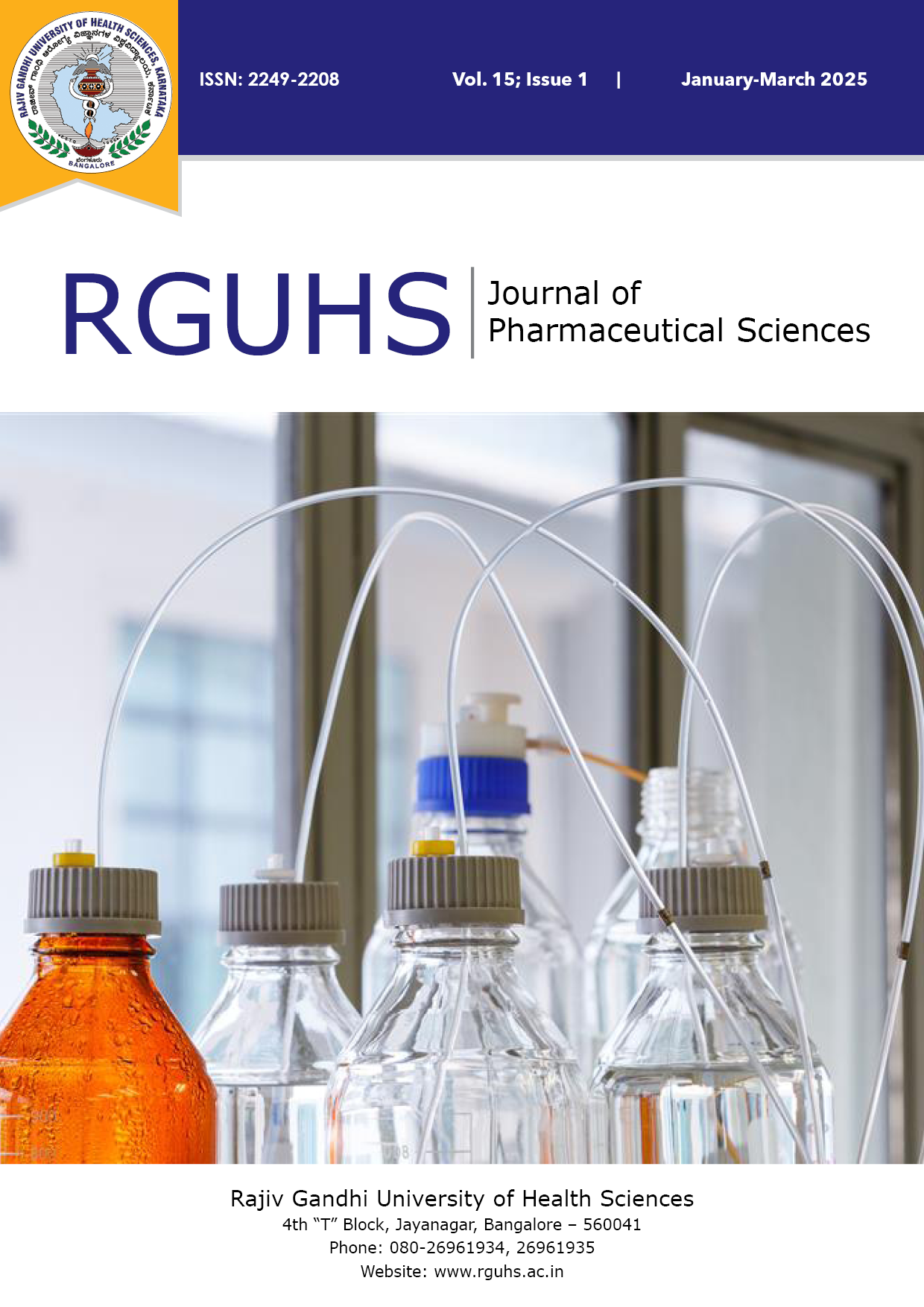
RJPS Vol No: 15 Issue No: 1 eISSN: pISSN:2249-2208
Dear Authors,
We invite you to watch this comprehensive video guide on the process of submitting your article online. This video will provide you with step-by-step instructions to ensure a smooth and successful submission.
Thank you for your attention and cooperation.
1Department of Pharmaceutics, Sree Siddaganga College of Pharmacy, B H Road, Tumakuru, Karnataka, India.
2Associate Professor, Department of Pharmaceutics, Sree Siddaganga College of Pharmacy, B H Road, Tumakuru, Karnataka, India.
3Department of Pharmaceutics, Sree Siddaganga College of Pharmacy, B H Road, Tumakuru, Karnataka, India.
4Department of Pharmaceutics, Sree Siddaganga College of Pharmacy, B H Road, Tumakuru, Karnataka, India.
*Corresponding Author:
Associate Professor, Department of Pharmaceutics, Sree Siddaganga College of Pharmacy, B H Road, Tumakuru, Karnataka, India., Email: ashokkumarscp@gmail.comDepartment of Pharmaceutics, Sree Siddaganga College of Pharmacy, B H Road, Tumakuru, Karnataka, India., Email:
Abstract
Objective: The major goal of the current study was to create and assess solid lipids nanoparticles of Hydralazine hydrochloride to improve its bioavailability.
Methodology: Solid lipid nanoparticles (SLN) have recently received a lot of media interest.. Researchers are interested in it because of its increased stability and lower toxicity. Thermal homogenization of hydralazine chlorhydrate and various lipids resulted in the formation of solid lipid nanoparticles. Tristearin, Glycerol mono stearate, and Compritol 888 were the lipids used, soy lecithin was utilized as a surfactant & stabilizer and tween 80, poloxamer-188 were used for regulated release. Drug release and properties followed a diffusion-controlled release pattern.
Results: Particle size and PDI (polydispersity index), zeta potential, entrapment efficiency, and in vitro drug release were all tested for the nanoparticles. The particle sizes varied between 45.72 and 576.4 nm. All the formulations had good PDI values ranging from 0.119 to 0.411. Blank SLN had a zeta potential of -15.2 mV, but drug-loaded SLN had a zeta potential ranging from -12.3 to -32.3 mV. The measured entrapment efficiency was in the range of 78.68% to 96.25%.
Conclusion: The cumulative percentage release of Hydralazine Hcl from different Hydralazine Hcl nanoparticles varied from 53.38% to 89.74% depending on the drug lipid ratio and the type of lipid used. The average percentage of drug released from different SLNs after 24 hours was in the following order: F9 (53.95%) < F6 (56.75%) < F4 (61.74%) < F7 (63.90%) < F5 (67.78%) < F8 (69.09%) < F3 (75.31%) < F1 (79.36%) < F2 (89.74%). The release kinetic studies showed that the release was first order diffusion controlled and the n values obtained from the Korsmeyer-Peppas model revealed the release mechanism was Quasi-Fickian type (n-value of 0.47).
Keywords
Downloads
-
1FullTextPDF
Article
Introduction
Solid lipid nanoparticles (SLN), first introduced in 1991, is an alternate carrier system to classic colloidal systems such as emulsions, liposomes, and polymeric micro and nanoparticles. SLNs are gaining popularity as new colloidal medication carrier for intravenous use. SLNs are submicron colloidal carriers ranging in size from 50 to 1000 nm and are made up of physiological lipid dispersed in water or aqueous surfactant solution. SLNs have unique qualities such as tiny size, large surface area, high drug loading, and phase interaction at the interface, and are appealing due to their potential to increase pharmaceutical efficacy.1
Anti-hypertensive drugs2
Antihypertensive medicines are a group of compounds that have the therapeutic target of preventing, regulating, or treating hypertension. Antihypertensive medication types differ structurally and therapeutically. They are important in anesthetic practice because they are commonly prescribed to general population, with an overall prevalence of hypertension in the UK being 31% [defined by the National Institute for Health and Care Excellence (NICE) as a measurement of 140/90 mm Hg or higher in clinic, followed by ambulatory or home measurement of 135/85 mm Hg or higher]. Antihypertensive medications are widely used in unrelated illnesses, such as thyrotoxicosis and anxiety, or angiotensin-converting enzyme inhibitors (ACEIs) in heart failure. As a result, both medicine and its indications are relevant to the administration of anesthesia.
The goal of this study was to create and test Hydralazine hydrochloride drug-loaded solid lipid nanoparticles made from tristearin, glycerol monostearate, and Compritol.
Materials and Methods
Hydralazine hydrochloride was acquired from Yarrow chemicals, Mumbai, Tristerin from Sasol Germany, Glycerol mono stearate (GMS) from Research-Lab Fine chem. Industries, Compritol from Gattefosse-France, Soy lecithin was obtained from Hi Media Laboratories Pvt. Ltd, and Tween 80, chloroform and methanol were purchased from SD Fine-Chem limited. All the reagents used were of analytical grade.
Fourier-transform infrared spectroscopy (FT-IR) FTIR spectroscopy was used to investigate drug-polymer interactions. FTIR analysis was performed on pure drug, excipients, and a physical combination of drug and excipients. The spectra were obtained by scanning an FTIR spectrophotometer in the wavelength range 400-4000 cm-1. 3 FT-IR analysis was performed on the following samples: a. Pure drug (Hydralazine hydrochloride), b. Physical combination of drug + Tristearin (1:1), c. Drug + GMS physical mixture (1:1). d. Drug + Compritol physical combination (1:1).
Preparation of solid lipid nanoparticles with Hydralazine HCL using lipids (Compritol, Tristearin and GMS)
Solid lipid nanoparticles were prepared by using lipid (Compritol, Tristearin and GMS). The lipid was first melted in a boiling tube using a water bath and then the soya lecithin and drug were incorporated into the melted lipid. Continued heating was done till the compounds in the boiling tube melted. If they did not melt, organic solvents like methanol and chloroform in the ratio 1:1 were added and heating was continued until all the organic solvent evaporates. Simultaneously in another beaker, tween 80 was taken and dissolved in water (aqueous phase) and heated to temperature equal to that of lipid phase. Then the aqueous phase was transferred slowly into the lipid phase while homogenizing the mixture at 20,000 rpm for five minutes under high-speed homogenizer and then immediately placed in probe ultrasonicator at 75% amplitude for 20 min. Temperature was maintained above 5°C of melting point of lipid during preparation. Blank nanoparticles were prepared similarly omitting the Hydralazine hydrochloride in the preparation.
DRUG- Hydralazine hydrochloride; TS = Tristearin; GMS=Glycerol Mono Stearate; CM = Compritol; Tw = Tween 80; Soy = Soy lecithin; DM H2O = Distilled Water
Characterization of Solid Lipid Nanoparticles
Particle size analysis
The particle size was estimated via dynamic light scattering on a Malvern zetasizer using vertically polarized light provided by an argon-ion laser (Cyonics). Experiments were carried out at a temperature of 25.0±0.1ºC and a measurement angle of 900 to the incident beam.4
Polydispersity index
Polydispersity index is a parameter calculated from a Cumulants analysis of the DLS-measured intensity auto correlation function. Polydispersity index was determined by the same instrument i.e., Malvern zetasizer.4
Zeta potential
Nanoparticles were diluted with distilled water and put in a transparent, disposable zeta cell at 25°C to measure zeta potential using a Malvern zetasizer. To establish the sample’s size and potential, two zeta runs were performed on it.5
Drug content
In the centrifuge tube, 0.2 mL of drug-loaded SLNs was added to 5 mL of methanol. After vortexed for 10 minutes, the solution was centrifuged at 5000 rpm for 30 minutes. The supernatant was collected. The drug content in the supernatant was determined using a UV spectrophotometer at 264 nm for Hydralazine hydrochloride.6
Drug content was calculated using the following formula.
% Drug content= Practical amount of the drug obtained / Theroretical amount of drug added
Percentage drug entrapment efficiency (%DEE)
About 2 mL of Hydralazine hydrochloride loaded solid lipid nanoparticles were obtained and placed in the outer chamber of centrisart device, with the sample recovery chamber placed on top of the sample. For 20 minutes, the unit was centrifuged at 5000 rpm. The solid lipid nanoparticles and encapsulated drug remained in the outer chamber, while the aqueous phase was transferred to the sample recovery chamber via a filter membrane (molecular weight cut-off 20,000 Daltons). The resultant aqueous phase was tested for Hydralazine hydrochloride at 264 nm using a UV-spectrophotometer. The following relationship was used to calculate the % Drug entrapment efficiency7
% Entrapment Efficiency = Total amount of drug - Amount of drug presnt in aqueous phase / Total amount of drug× 100
In vitro drug release studies
The Franz diffusion cell was used for in vitro drug release research. For the diffusion investigation, 2 mL of nanoparticles dispersion was employed. The donor compartment was filled with drug-containing nanoparticles, while the receiver compartment was filled with 22 mL of diffusion medium Phosphate buffer pH 6.8 kept at room temperature in a Franz diffusion cell. The magnetic bead’s rotational speed was kept constant at 50 rpm. At predetermined intervals, 1 mL of the aliquot was removed. A UV spectrophotometer set to 261 nm was used to determine the drug content of the samples. To maintain the sink state, an equal volume of the diffusion medium was supplied in the vessel after each withdrawal. For each formulation, three trials were conducted. The percentage drug release was calculated and displayed against time as a function of data to analyze the pattern of drug release.8
Results
Determination of λmax of Hydralazine hydrochloride was carried out in methanol and phosphate buffer of pH 6.8.
A solution of Hydralazine hydrochloride was scanned in UV spectrophotometer between 200-400 nm. Hydralazine hydrochloride showed absorbance max at 264 nm for methanol and 261 nm for phosphate buffer pH 6.8.
Hydralazine hydrochloride standard graph in methanol
Hydralazine hydrochloride standard solution in methanol (2-16 g/mL) was produced and measured at 264 nm using a UV spectrophotometer. Figure 1 shows the typical plot of Hydralazine hydrochloride.
Hydralazine hydrochloride standard plot in phosphate buffer
Standard solutions of Hydralazine hydrochloride (2- 18 g/mL) in phosphate buffer at pH 6.8 were produced and measured at 261 nm with a UV spectrophotometer. Hydralazine hydrochloride standard plot is presented in Figure 2.
FT-IR studies
Separately, FT-IR spectroscopy analysis was performed to determine the compatibility of the medicine, Hydralazine Hcl and the lipids (TS, GMS, and CM utilized in the manufacture of nanoparticles. The FT-IR analysis was carried out on drug, lipids, surfactants, and a physical combination of drug and lipids. The FT-IR spectroscopy spectra at wave numbers ranging from 400-4000 cm-1 are displayed below.
Zeta potential, size, and PDI (Polydispersity index)
Particle size, PDI, and zeta potential were measured in blank and Hydralazine hydrochloride SLNs produced with lipids (Tristearin, Compritol, and GMS) w/v using tween 80 (Table 4). Figures 7 and 8 illustrate the size report and zeta potential obtained from the zeta size. Blank nanoparticles had a diameter of 167.1 nm, but drug-loaded particles had a diameter of 45.72 to 576.4 nm. All formulations had good PDI values ranging from 0.119 to 0.658. Blank SLN had a zeta potential of -10.4 mV, but drug-loaded SLN had a zeta potential of -10.4 mV to -32.3 mV. Similarly, Hydralazine hydrochloride loaded SLNs of formulation F2 had particle sizes range of 56.70 d. nm and PDI of 0.332 and Zeta Potential of -32.3 mV. The sizes were in the nano range, and the resultant zeta potential was optimal for excellent stability.
Drug content
The drug content of formulations was carried out by extraction with methanol as mentioned in the methodology section. Formulations (0.2 mL) were extracted from Hydralazine Hcl using 5 mL of methanol. The drug content results ranged between 94.35% to 99.05%.
Entrapment efficiency (EE)
In formulations made with solid lipids, the average entrapment effectiveness of Hydralazine Hcl SLNs was reported to be in the range of 78.68% to 96.25%. They were lipophilic (Log P value 5.06) and virtually insoluble in water. As a result, medication partitioned advantageously towards melted lipids during homogenization and sonication at high temperatures. As a result, the enhanced formulation F2 had a better percentage entrapment efficiency of 92.89%.
Release studies
The drug release from nanoparticles was investigated using the Franz diffusion method. Tables 4 and 5 illustrate the in vitro release characteristics of Hydralazine Hcl from Hydralazine Hcl nanoparticles. Depending on the drug lipid ratio and the type of lipid utilized, the cumulative percentage release of Hydralazine from distinct Hydralazine Hcl nanoparticles ranged from 53.95% to 89.74%.
In vitro release data were fitted to various kinetic equations, including zero order, first order, the Higuchi model, and the Korsmeyer-Peppas model. Table 9 and Figures 12 to 15 demonstrate a model processing of the F2 in vitro release. A similar method was used for the remaining formulation.
Discussion
In the present study, an attempt was made to develop solid lipid nano particulate delivery system for lipophilic drug Hydralazine Hcl using Tristearin, GMS and Compritol as carrier matrices, which are meant to be used for better anti-psychotic action. FT-IR experiments were performed to determine the potential interaction between the selected medication and lipids. FT-IR experiments found no interaction between the chosen medication and lipids. Solid lipid hydralazine Hcl, the heat homogenization process was used to create nanoparticles. The approach produced nanoparticles with acceptable range and stability. All of the formulations had extremely high entrapment efficiency. SLNs were created by combining 0.5 to 1.5% w/v lipids. In the case of F2, lipid and surfactant were tuned after taking particle size, zeta potential, and in vitro drug release profile into account.
The size, PDI, and zeta potential of the generated F1 to F5 formulations were acceptable and adequate. Entrapment efficiency of F1 to F5 formulations was found to be greater than 70% on an average. The average entrapment efficiency of most Hydralazine Hcl SLNs was better than 80%, with the optimized formulation F2 showing 92.89% entrapment. Hydralazine Hcl release from nanoparticles was governed by Quasi-Fickian diffusion, according to release kinetics studies.
Conclusion
From our findings, it is possible to infer that the lipid nanoparticle delivery system of Hydralazine Hcl developed utilizing generally acknowledged and physiologically safe lipids was capable of demonstrating sustained release qualities for a period of 24 hours. They may therefore minimize the frequency of dose, reducing the occurrence of side effects, improving dissolution rate, and increasing the drug’s effectiveness.
Conflict of interest
None
Acknowledgement
The authors are thankful to the administration, Sree Siddaganga College of Pharmacy, Tumakuru for giving essential facilities to do this work and make it fruitful.
Supporting File
References
- Havanoor SM, Manjunath K, Bhagawati ST, Veerapur VP. Isradipine loaded solid lipid nanoparticles for better treatment of hypertension– preparation, characterization and in vivo evaluation. Int J Biopharm 2014;5:218-24.
- Creeth HD, Rees E, Legge SE, Dennison CA, Holmans P, Walters JT, et al. Ultra rare coding variants and cognitive function in schizophrenia. JAMA Psychiatry 2022;79(10):963-70.
- Shah M, Agrawal YK, Garala K, Ramkishan A. Solid lipid nanoparticles of a water soluble drug,ciprofloxacin hydrochloride. Indian J Pharm Sci 2012;74(5):434.
- Fissan H, Ristig S, Kaminski H, Asbach C, Epple M. Comparison of different characterization methods for nanoparticle dispersions before and after aerosolization. Anal Methods 2014;6(18):7324-34.
- Singh S, Dobhal AK, Jain A, Pandit JK, Chakraborty S. Formulation and evaluation of solid lipid nanoparticles of a water soluble drug: zidovudine. Chem Pharm Bull 2010;58(5):650-5
- Gouda R, Baishya H, Qing Z. Application of mathematical models in drug release kinetics of carbidopa and levodopa ER tablets. J Dev Drugs 2017;6(02):1-8.
- Cirri M, Maestrini L, Maestrelli F, Mennini N, Mura P, Ghelardini C, et al. Design, characterization and in vivo evaluation of nanostructured lipid carriers (NLC) as a new drug delivery system for hydrochlorothiazide oral administration in pediatric therapy. Drug Deliv 2018;25(1):1910-21.
- Manjunath K, Venkateswarlu V. Pharmacokinetics, tissue distribution and bioavailability of nitrendipine solid lipid nanoparticles after intravenous and intraduodenal administration. J Drug Target 2006;14(9):632-45